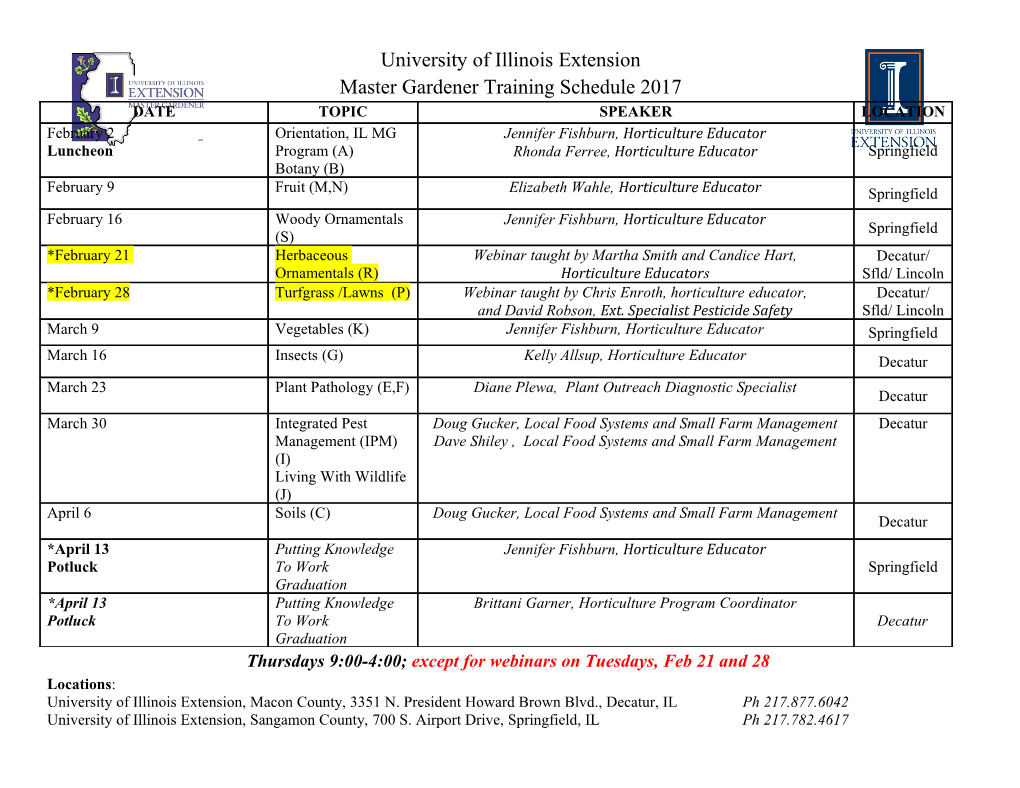
Measurements of the maximum depth of air shower profiles at LHC energies with the High-Elevation Auger Telescopes Zur Erlangung des akademischen Grades eines Doktors der Naturwissenschaften an der Fakultät für Physik des Karlsruher Instituts für Technologie (KIT) genehmigte Dissertation von Dipl.-Phys. Alaa Metwaly Kuotb Awad aus El Fayoum/Egypt Tag der mündlichen Prüfung: 21.12.2018 Referent: Prof. Dr. Dr. h.c. Johannes Blümer Korreferent: Prof. Dr. Ralph Engel Betreuer: Dr. Ralf Ulrich ii Abstract More than 100 years after their discovery, the nature of cosmic rays is still a mystery in many aspects. The subject of this thesis is to measure the mass composition of the cosmic rays at an energy range from 1015.8eV to 1017eV. This relies on the Cherenkov light emitted in the forward direction of the shower, directly pointing towards the telescopes. A new technique was proposed for the reconstruction named Profile Constrained Geometry Fit (PCGF). The benefit of this special technique is a high accuracy geometry reconstruction, which is pos- sible using only the telescope signals. A full PCGF dataset is produced for the Cherenkov dominated showers observed by the High Elevation Auger Telescopes (HEAT). The mass composition is deduced from the distribution of the maximum depth of those showers, Xmax. The first two moments of the distribution, hXmaxi, and s(Xmax), are compared to their counterparts of proton and iron simulations. The performance of the reconstruction and the analysis is studied using a complete time-dependent Monte Carlo simulation. It is a novel technique that is used for the first time in Pierre Auger, and by which the mass composition is measured in the energy region where there are signatures of cosmic ray transition from galactic to an extragalactic origin. Successfully, the Pierre Auger measurements in this as- pect are extended by one order of magnitude to lower energy toward the knee. The results showed an interesting composition that is heavily dominated by iron around the second knee and towards the knee, it starts to get lighter. The measurements in this energy region will play a critical role in understanding key features of the cosmic ray nature. iii iv Contents 1 Introduction1 2 Cosmic rays3 2.1 Energy spectrum . .3 2.2 Sources of cosmic rays, acceleration and propogation . .4 2.2.1 Acceleration mechanism . .4 2.2.2 Propagation . .6 2.2.3 Sources . .6 2.3 Transition from galactic to extragalactic . .7 2.4 Extensive air showers . .8 2.4.1 Electromagnetic cascade . 10 2.4.2 Hadronic cascade . 10 2.5 Light production . 13 2.5.1 Fluorescence light . 13 2.5.2 Cherenkov light . 13 2.6 Air shower simulations . 14 2.7 Mass composition from air shower observables . 15 3 Pierre Auger Observatory 19 3.1 Surface detector . 19 3.2 Fluoresence detector . 20 3.2.1 The telescopes . 20 3.2.2 The High Elevation Auger Telescopes . 22 3.2.3 Trigger and data acquisition system . 26 3.2.4 Calibration . 26 3.2.5 Atmospheric monitoring . 27 3.3 Reconstruction of telescope data . 28 3.3.1 Geometrical reconstruction . 29 3.3.2 Reconstruction of the longitudinal profile and energy . 30 3.4 Profile Constrained Geometry Fit . 31 4 HEAT-Coihueco cross-calibration 35 4.1 HEAT-Coihueco virtual eye . 35 4.1.1 HEAT campaigns in downward mode . 36 4.2 Event selection and procedures . 36 v vi CONTENTS 4.3 Coihueco versus HEAT-downward results . 39 4.4 Coihueco versus HEAT-downward from PCGF aspect . 43 5 Testing the performance of analytical Cherenkov light model in Offline 45 5.1 Simulations of Cherenkov showers with CORSIKA ................ 45 5.1.1 CORSIKA configuration . 45 5.1.2 Shower geometry generation . 47 5.2 Results . 48 5.2.1 Shower detector plane . 49 5.2.2 Shower axis . 50 5.2.3 Shower parameters Xmax and energy . 50 6 Event reconstruction and selection 59 6.1 Data production . 59 6.2 Event Selection . 59 6.2.1 The basics selections . 60 6.2.2 The quality selections . 62 6.3 Field of view . 63 6.3.1 Geometrical field of view . 64 6.3.2 Expected field of view . 65 6.3.3 Fiducial field of view . 67 7 The analysis performance with RealMC simulation 75 7.1 RealMC library . 75 7.2 Biases and resolution of Cherenkov dominated showers . 76 7.2.1 Testing the energy reconstruction . 78 7.2.2 Testing Xmax reconstruction . 79 7.3 End-to-end analysis cross-check . 82 8 Stability and systematic uncertainty of Xmax 87 8.1 Stability of hXmaxi ................................... 87 8.2 Data correction . 90 8.3 Systematic uncertainties . 90 8.3.1 Energy uncertainty . 91 8.3.2 Systematic uncertainties of Xmax and resolution . 91 8.3.3 Total systematic uncertainties . 93 9 Results 97 9.1 Moments of Xmax ................................... 97 9.1.1 The mean depth of the shower maximum . 97 9.1.2 Fluctuations of the depth of shower maximum . 98 9.2 Comparison to other cosmic ray experiments . 99 9.3 Average logarithmic mass . 101 9.4 Implications for proton-air cross section measurement . 103 10 Summary 107 A Offline configurations 127 BCORSIKA 131 B.1 CORSIKA steering card . 131 B.2 HEAT full CORSIKA simulation and reconstruction . 132 CONTENTS vii C Cut list 137 C.1 General cuts . 137 C.2 Geometry and profile cuts . 138 D Calculation of truncated mean of Xmax 139 E Field of view 141 E.1 The expected FoV . 141 E.2 Fiducial FoV . 142 E.2.1 Data fiducial FoV . 143 E.2.2 Monte Carlo fiducial FoV . 144 F Xmax distributions 145 G Data and Monte Carlo shower geometry investigation 147 G.1 Rp distributions . 147 G.2 Zenith distributions . 148 viii CONTENTS CHAPTER 1 Introduction The research of “Cosmic Rays” first appeared in the scope of physics in 1912 [1]. Today cosmic rays are understood to be as ionized particles bombarding the Earth from outer space, having energies varying at many order of magnitude of energy from a few GeV to more than 100 EeV. The record in energy measured so far is about 3 × 1020eV, which was observed by Fly’s Eye cosmic ray detector in 1991. If this highest energy particlep was a proton, then the energy of its interaction with an air nucleus would correspond to s ≈ 750TeV. Countless theories have been proposed to explain the origin of ultra high energy cosmic rays (UHECRs). Some of them rely on a new physics, and others are more conventional, based on ordinary matter undergoing an acceleration process in a magnetic field of an astro- physical system. Actually, our understanding of the cosmic rays has improve exponentially thanks to modern reliable experiments built especially for this task. It is one of the big questions of fundamental physics to find the origin of cosmic rays that in turn will offer more understanding of the universe around us. One of these experiments, which proudly I am a member of its collaboration, is the Pierre Auger Observatory. Pierre Auger Obser- vatory recently found that the cosmic rays at energies above 8 EeV are propagated to us from extragalactic sources [2]. On the other hand, it is well known that the cosmic rays with energies up to 10 PeV originate inside our galaxy. According to this, there must be a tran- sition between 10 PeV and 8 EeV with a change of the cosmic ray sources. The nature of this transition is one of the biggest mysteries of cosmic rays research today. Many scenarios predict a changing in the mass composition from heavy to light at the transition region. The preference between those models requires accurate measurements of the mass composition in this energy region. One of those scenarios, the “ankle model”, which predicts a heavy composition around the ankle region, was already disfavored after the measurements of the Pierre Auger Observatory [3], and Telescope Array [4] showing that the composition is light around (3 − 5) × 1018eV. So indeed the composition study is of major importance along the whole range of cosmic rays energies. Understanding the mass composition of cosmic rays in the low energy region of the UHECR spectrum will represent a crucial piece of information, which is needed for a break- through in the UHECR physics, since this will put constraints on possible sources and accel- eration mechanisms. I am proud to present this thesis as a piece in solving this puzzle. In this thesis, I measure how the cosmic ray compositions change as a signature of the transition from galactic to extragalactic sources. This is the first step by the Pierre Auger Observatory to measure the mass composition at such low energies. It was necessary to make use of a dif- ferent and custom-developed reconstruction approach for the data analysis. An introduction 1 2 Chapter 1. Introduction to cosmic rays and the derivation of their mass composition from air shower observables is presented in Chapter2. At energies above ≈ 1018eV, the Pierre Auger uses a hybrid design to observe the UHECR. It consist of a large surface array (SD) of 1660 water-Cherenkov tanks covering 3000 km2 overlooked by 24 fluorescence telescopes (FD) in four sites enclosing the boundary of the SD. To extend the hybrid observation energy down to 1017eV the three High Eleva- tion Auger Telescopes (HEAT) were added to FD of Pierre Auger at the Coiheuco site. Only the showers observed by HEAT are directly involved in the final result of this analysis by concentrating mainly on the showers induced from the Cherenkov light. Performing the analysis in the energy range of interest, (1015.8 − 1017)eV, is not possible with the Pierre Auger standard hybrid technique of reconstruction.
Details
-
File Typepdf
-
Upload Time-
-
Content LanguagesEnglish
-
Upload UserAnonymous/Not logged-in
-
File Pages159 Page
-
File Size-