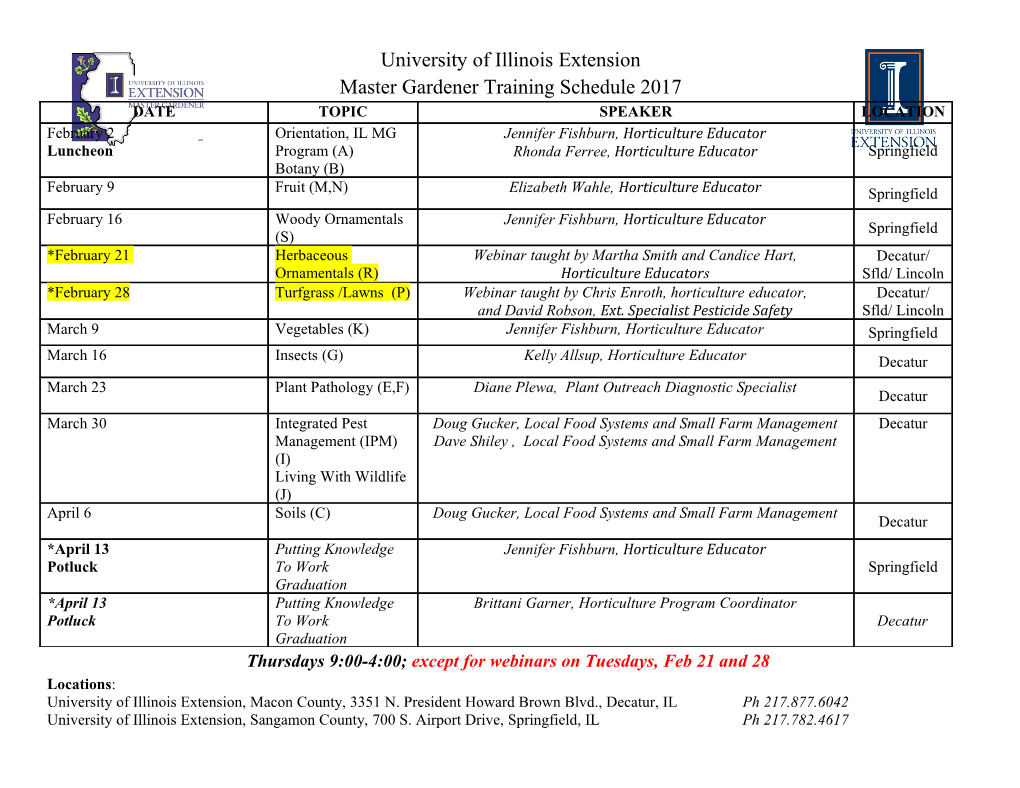
Introduction to Cosmology (essentials of the lectures) F. Jegerlehner Humboldt University, Berlin – summer 2011 – 0-0 General Setup Cosmology = theory of universe at large ( > 1 Gigaparsec ) ∼ Content = pressure free “dilute gas of galaxies”, cosmic microwave background radiation, etc.: electromagnetically neutral stuff ➠ gravity only Dynamics = Gravity ➠ Einstein’s General Relativity Theory Cosmology’s metric space: assuming cosmological principle and Weyl’s postulate ➠ Comoving coordinates = distinguished cosmological frame of reference in which matter is at rest F. Jegerlehner Humboldt University, Berlin 1 Robertson-Walker Metric the Metric of Cosmology Cosmological principle: the cosmos looks homogeneous and isotropic spatial part must be 3–dimensional space of ⇒ constant curvature. Case of positive curvature can be viewed as a sphere in a 4–dimensional space: 2 2 2 2 x1 + x2 + x3 + x4 = 1/k = constant x2 = k ~x 2 = 1/k r2 2x dx = 2rdr 4 − − ⇒ 4 4 − with r = ~x . In spherical coordinates: | | (x ,x ,x ) (r, θ, ϕ) yields 1 2 3 → 2 2 2 2 2 dℓ = dx1 + dx2 + dx3 + dx4 r2 dr2 = dr2 + r2 (dθ2 + sin2 θdϕ2) + 1/k r2 − dr2 = + r2 (dθ2 + sin2 θdϕ2) 1 kr2 − We can rescale this spatial line elements with the factor R2 and restrict k to values 0, 1: ± dr2 dℓ2 = R2 + r2 (dθ2 + sin2 θdϕ2) 1 kr2 − then Rr = physical radius, r = coordinate radius and the space has curvature k/R2. F. Jegerlehner Humboldt University, Berlin 2 The parameter k characterizes the type of the geometry of the spaces of constant curvature Type Geometry Curvature k = +1 spherical positive k = 0 euclidean no (flat) k = 1 hyperbolica negative − ❒ Cosmological principle + Weyl’s postulate (stuff in universe follows hydrodynamical flow) ➠ the complete Robertson-Walker line element then may be written as dr2 ds2 = c2dt2 R2(t) + r2 (dθ2 + sin2 θdϕ2) − 1 kr2 − Determines all measurements of space and time in the universe! to every point there exists a local rest frame: • co-moving system, co-moving coordinates co-moving observer measures t as Eigentime • t is called the cosmic time • ρ(P ): total energy density • p(P ): isotropic pressure in the co-moving system only depend on t (cosmological principle, Weyl postulate) F. Jegerlehner Humboldt University, Berlin 3 Propagation of light and spectral red shift: d2s =0 in radial direction: dθ =0; dϕ =0 cdt = dr R(t) √1 kr2 → − Spectral ratio: . ζ =1+ z = ν1 =1+ ∆λ ; ∆λ = c (∆t ∆t ) ν0 λ1 0 − 1 z is the spectral shift: red shift ∆λ z > 0 z = λ1 z < 0 blue shift r1 t0 1 dr = dt c √1 k r2 S(t) r0=0 − t1 R R independent of t. The r integral yields r arcsin r ; k = 1 dr′ = r ; k = 0 √ 2 1 kr′ Z0 − arcsinh r ; k = 1 − Red shift: ∆t0 ∆t1 ∆t0 S(t0) S(t0) = S(t1) hence ζ =1+ z = ∆t1 = S(t1) Result: the time dependent scale factor S(t) implies a red shift z = S(t0) 1 S(t1) − F. Jegerlehner Humboldt University, Berlin 4 Distances and Hubble’s law: The coordinate distance between an event (t1,r1) and the observer (t0,r0 = 0) is r1. The proper distance D can be defined by setting dt =0: (τ = t t ) 1 0 − 1 r1 dr D1 = S(t0) √1 kr2 Z0 − r3 = S(t ) r + k 1 + 0 1 6 ··· t0 S(t ) = c dt 0 S(t) tZ1 τ τ 2 = cτ 1+ H + H2 (2 + q )+ 2 0 6 0 0 ··· Inverting this relation, we obtain D H D 2 H2 D 3 τ = 1 0 1 + 0 (1 q ) 1 + c − 2 c 6 − 0 c ··· and the red shift is the given by 2 2 z = H D1 + H0 (1 + q ) D1 + 0 c 2 0 c ··· Here, D1 is the present distance (observation time) of the galaxy. The form of the relation exhibits the two key parameters H0 and q0 and D1 z = H0 c is the Hubble law. The latter would be valid as an exact relation if the expansion would be F. Jegerlehner Humboldt University, Berlin 5 non-accelerated: q0 =0 such that S(t)= S(t ) (1+(t t ) H ) ; q =0 0 − 0 0 0 Depending on the value of q0 we have: q > 0 the expansion is decelerated • 0 q < 0 the expansion is accelerated • 0 Observed: first by Hubble 1929: Present status: H = 74.2 3.6 (km/s)/Mpc [HST 2009] 0 ± In 1968, the first good estimate of H0, 75 km/s/Mpc, was published by Allan Sandage, but it would be decades before a consensus was achieved. Proved universal expansion!!! Measuring S˙(t0) as well as S¨(t0): F. Jegerlehner Humboldt University, Berlin 6 S˙(t0) Hubble constant H0 = H(t0)= S(t0) S¨(t0) S(t0) q0 = q(t0)= 2 deceleration parameter − (S˙(t0)) A closer look: deviation from linear relation, from observations one presently extracts: H0 = 74.2 ± 3.6 km/sMpc(HST) 71 ± 4 km/sMpc (WMAP) q0 = −0.60 ± 0.02 Type Ia super nova reveal an accelerated expansion of our universe Requires positive cosmological constant (Λ > 0)! Proves accelerated expansion!!! Supported by power spectrum of CMB fluctuations! Note: Cosmological constant not so important for evolution of universe in the F. Jegerlehner Humboldt University, Berlin 7 past, but determines its future! (see Freidmann’s equations) For q0 =0 the age of the universe is 1 9 ● H− = (13.7 0.2) 10 years [Hubble age] 0 ± · the time back a Big Bang must have been taking place. The corresponding horizon of the universe, where the escape velocity reaches the speed of light c is ● D = c 3.34 Gpc max H0 ≃ about 10.9 109 light years. · Note: observable Cosmology commences at > 1 Gpc and ends at the horizon 3.34 Gpc. ∼ F. Jegerlehner Humboldt University, Berlin 8 Friedmann equation Homogeneous, isotropic matter distribution: Einstein’s field equations Friedmann’s solution ⇒ 2 ˙ 2 2 R 8πGρtot c H = = k 2 R! 3 − R G Gravitational constant ρtot Total energy-matter density R(t) Radius of the universe R˙ (t) Expansion rate (R˙ dR/dt) ≡ k 0, 1 Type of geometry ± Cosmological constant Λ: Λ ρ + ρ ρ ; ρ = tot|Λ=0 Vacuum→ tot Vacuum 8πG For simplicity we take: Λ = 0 (was not relevant in past, just starts to be relevant) Observations require Λ > 0 (dark energy)! F. Jegerlehner Humboldt University, Berlin 9 Friedmann’s equation: derivation v R Energy balance: per unit mass (mass in spherical shell = 4πR2dR ρ) Kinetic energy + potential energy = constant 1 G M(R) v2 = constant 2 − R 4π M(R)= R3 ρ, v = dR/dt R˙ 3 ≡ yields, after division by R2 and multiplication with 2, 2 R˙ 8πG ρ c2 = k R − 3 − R2 ! F. Jegerlehner Humboldt University, Berlin 10 Basic dynamical equations of Cosmology R˙ 2 = Λ R2 + 8πG ρR2 kc2 : Friedmann equation 3 3 − d (ρR3) = 3p R2 : Energy balance dR − p = p(ρ) : Equation of state The equation of state of matter and radiation is determined by the thermodynamic properties of the energy distribution: gas, liquid, solid, dust, radiation. For the state of matter electromagnetic, weak and strong interaction forces play the key role. Of interest for cosmology: Radiation: 1 2 dominates at very high temperatures p = 3 ρc Matter pressure-free: p = 0 after cooling down and dilution by expansion Vacuum energy: p = ρc2 after cooling in future becomes dominating term − F. Jegerlehner Humboldt University, Berlin 11 Observable quantities: Curvature : K(t) = k/R2(t) Hubble constant : H(t) = R˙ (t)/R(t) Deceleration parameter : q(t) = (R¨(t) R(t))/R˙ 2(t) − Friedmann equation: 2 κ H (t) = 2 K(t) 2 (ρ(t) + p(t)) c (q(t)+1) − 2 κ H (t) λ = (ρ(t) + 3p(t)) 3 2 q(t) 2 − c 2 8πG ( , 2 ) λ = Λ/c κ = 3c F. Jegerlehner Humboldt University, Berlin 12 Matter dominated universe Universe as a gas cloud of galaxies Non-relativistic gas, kinetic energy negligible relative to rest mass: dust p 0 ∼ Density of non-relativistic particles is proportional to 1/R3 3 3 ρ(t) R (t) = ρ0 R0 = constant R˙ 2 κ C = ρ R2 k = mat k c2 3 − R − κ C ρ R3 = constant mat ≡ 3 elementary integrable k = 1: R(t)= Cmat (1 cos η), ct = Cmat (η sin η) 2 − 2 − 9 1/3 2/3 k = 0: R(t)=( 4 Cmat) (ct) k = 1: R(t)= Cmat (cosh η 1), ct = Cmat (sinh η η) − 2 − 2 − Behavior for small t: 9 R(t) ( C )1/3 (ct)2/3 , k = 0, 1 ≃ 4 mat ± independent of k ! all three cases: Big-Bang solutions Cold “Big-Bang” !!! F. Jegerlehner Humboldt University, Berlin 13 Behavior for large t: k = 1: cyclic, cycle ctc = πCmat 9 1/3 2/3 k = 0: R(t)=( 4 Cmat) (ct) k = 1: R(t) ct − ≃ The product M = ρR3 is constant and corresponds to the total mass. Galaxies, clusters of galaxies, dark matter (v c) ≪ F. Jegerlehner Humboldt University, Berlin 14 Einstein - de Sitter Universe k = 0 is the limiting case in between closed and open universe No curvature : K(t) 0 ≡ Deceleration parameter : qEdS(t) 1/2 ≡ 2 3 H (t) Matter density : 2 ρEdS(t) = κ c κ C ρ R3 mat ≡ 3 κ 3 H2(t) = R3 3 κ c2 κ 3 H2(t) 9 = C (ct)2 3 κ c2 4 mat Hence cosmic time is: 2 −1 t = 3 H (t) Today: H 0.52 10−28 cm−1 c 0 ∼ × × ρ 4.6 10−30gr/cm3 c2 0EdS ≃ × × − t = 2 H 1 13 109 years 0EdS 3 0 ≃ × Age of the universe if it would be flat! ⇒ (CMB says: universe is flat!) Generally: ρ>ρEdS if k = +1 ρ(t) = ρEdS(t) 2q(t) · ⇒ ( ρ<ρEdS if k = 1 − F.
Details
-
File Typepdf
-
Upload Time-
-
Content LanguagesEnglish
-
Upload UserAnonymous/Not logged-in
-
File Pages56 Page
-
File Size-