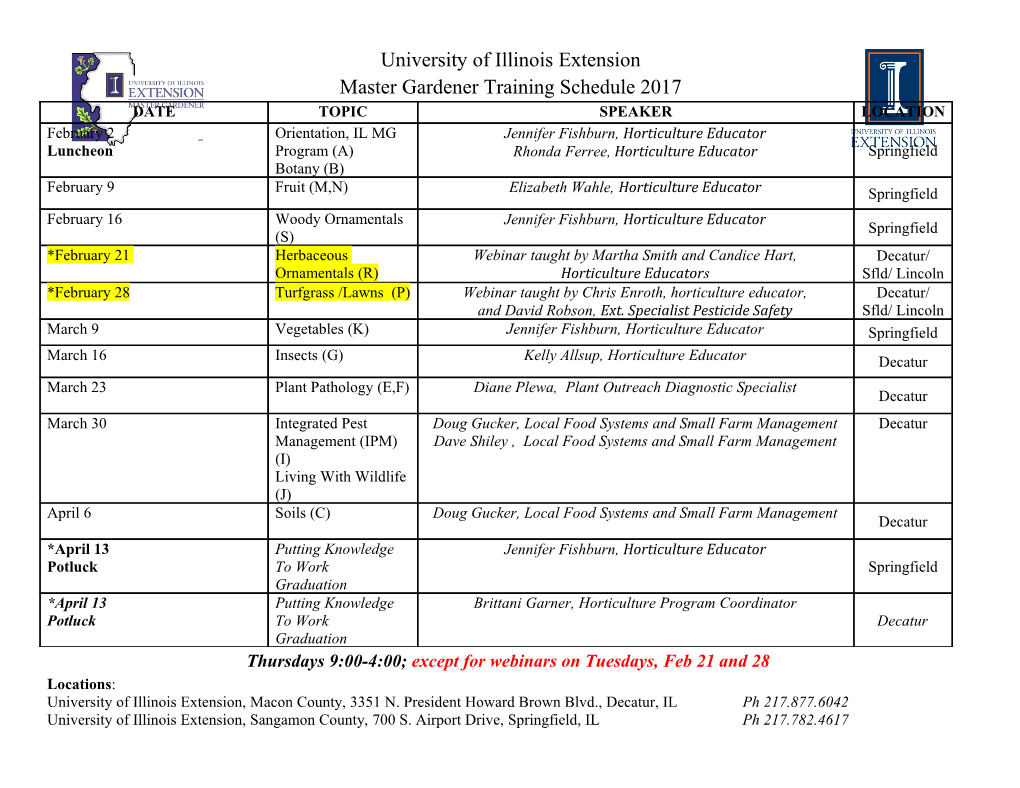
Journal of Electronic Materials, Vol. 21, No. 10, 1992 Effects of Illumination During Anodization of Porous Silicon C. TSAI, K.-H. LI, and J. C. CAMPBELL Microelectronics Research Center Department of Electrical and Computer Engineering The University of Texas at Austin, Austin, Texas 78712 B. K. HANCE, M. F. ARENDT, and J. M. WHITE S.-L. YAU and A. J. BARD Department of Chemistry and Biochemistry The University of Texas at Austin, Austin TX 78712 The effects of illumination during anodic etching of porous Si have been studied. For a fixed current density and anodization time, it has been observed that below a critical irradiance level, increasing the radiant flux density during anodization results in higher photoluminescence and a blue shift of the photoluminescence spectra. For irradiance above the critical value, the photoluminescence intensity decreases. Transmission Fou- rier-transform infrared spectroscopy, x-ray photoelectron spectroscopy and atomic force microscopy have been employed to investigate the effects of illumination on the char- acteristics of porous Si. Key words: Porous Si, photoluminescence, illumination effect INTRODUCTION these porous Si samples, repsectively. In addition, x-ray photoelectron spectroscopy (XPS) has been The realization of practical, Si-based light-emit- utilized to provide information on Si-Si and Si-O ting devices would have a significant impact on nu- binding energies (BE) and the relative atomic con- merous technologies including optoelectronic inte- centration of O, Si, F, and C. We conclude that the grated circuits, optical memories and logic, and level of irradiance during anodization effects the advanced display systems. Previously, however, Si average dimensions of the porous Si microstruc- has exhibited extremely weak emission owing to its tures, the concentration of Si hydride and Si oxide, indirect bandgap. Recent observations of efficient, and the types of chemical bonds that predominate room-temperature visible emission from porous Si 1- near the surface. These effects, in turn, influence 3 have stimulated research and extensive debate on the PL intensity and the position of the peak of the the physical mechanisms responsible for this lu- emission spectrum. minescence. To date, the explanations that have been proposed can be categorized as (1) two dimensional quantum confinement in the microstructure of highly EXPERIMENTAL PROCEDURE AND porous Si, 1'3-7 or (2) the formation of a wide-band- RESULTS gap material such as a-SiHx, s or siloxene9 during anodization. Recent work 1~ has shown that the photoluminescence (PL) intensity of porous Si sig- A. Sample Preparation nificantly decreases when the samples are annealed The porous Si samples were prepared on (100) ori- in vacuum at temperatures >350 ~ C. Transmission ented, boron-doped Si substrates (resistivity ~ 1/~cm) Fourier-transform infrared (FTIR) spectroscopy has using a Teflon sample holder 13 in contact with a shown that this decrease in PL intensity coincides copper anode. Through a one-inch-diameter circu- with the desorption of hydrogen from the Sill2 spe- lasr opening in the fixture, the portion of the wafer cies. Also, it has been observed that predominant to be anodized was exposed to an electrolyte, con- Sill termination results in weak photolumines- sisting of 2 parts deionized water, 1 part acetone, cence, whereas the PL intensity is recovered when and 1 part 49% HF. During anodization the wafer the Sill2 is restored. H The link between the PL in- was illuminated with a tungsten lamp that had its tensity and the Sill2 concentration is corroborated peak intensity at 620 nm. The irradiance was mea- in this paper, through a study of the PL spectra of sured with a Si power meter. During the 15 min porous Si samples formed by irradiance at various anodization process, the current was maintained at optical power levels during anodization. Transmis- 10 mA/cm2. The anodization procedure was re- sion FTIR and atomic force microscopy (AFM) were peated for irradiant flux levels of 2, 3, 4, 6, 8, and used to measure the concentration of the surface Si 10 mW. Visually, it appeared that the luminescence hydride species and to monitor the morphology of of as-formed porous Si samples, as monitored with excitation from a 365 nm UV lamp, peaked at shorter (Received April 9, 1992) wavelengths with increasing illumination power. 0361-5235/1992/1401-99555.00 TMS 995 996 Tsai, Li, Campbell, Hance, Arendt, White, Yau and Bard 40(} ' I ' I ' I ' I ' I ' 900 I I I I I I I I Si-Si Stretch Modes ,--, - ..... Wavelength (run) Si-O-Si Si',H2 .~ 30(3 " ~ ,-~ ,,.., [a A 2roW ~ /% 800 ~= LIJ 2o~ ~ 0 "~= Z <[ nO n- O (/) % m <[ .. smw 10 mW (~, i I , I , I I I I I 600 I l 1 I I I I I I 0 2 4 6 8 t0 12 2200 2000 1800 1600 1400 1200 1000 800 600 Illumination Level (roW) WAVENUMBER Fig. 1 -- Intensity and wavelength of maximum luminescence Fig. 2 -- Transmission FTIR spectra for illumination levels of 2, vs illumination level during anodic etching. 3, 4, 6, 8, and 10 mW. B. Photoluminescence Measurements with illumination from 2 to 6 mW but decreases for After the samples were removed from the anod- the high porosity 8 and 10 mW samples. On the other ization chamber and dried in air, PL spectra were hand, the Si-O-Si peak continues to increase with obtained with a 1-m scanning spectrometer and a illumination up to 10 mW, which indicates that more photomultiplier with a GaAs photocathode. The op- Si is oxidized for samples of higher optical powers. tical pump for the luminescence was an Ar laser (power = 10 mW, 1 = 488 nm). The laser beam was expanded to a diameter of 1 cm in order to avoid D. Atomic Force Microscopy photoassisted degradation of the PL intensity. ~e Figure 1 shows that the PL peak intensity and the The AFM used in this study was a commercial peak wavelength versus the illumination level dur- Nanoscope II (Digital Instrument Inc, Santa Bar- ing anodization. For illumination up to 6 mW, the bara, CA). It utilized microfabricated V-shaped Si PL intensity increases and the peak wavelength de- nitride cantilevers (100 /zm long, wide legs) onto creases. For irradiance >6 mW the PL intensity which sharp tips attached. Two scanners (15/xm and drops but the peak wavelength continues to de- 1.0 /xm) were able to achieve atomic resolution on crease. atomically flat substrates such as newly cleaved py- rolytic graphite and mica. However, for rough sam- C. Transmission Fourier-transform Spectroscopy ples such as the porous Si, the smaller scanner pro- vided better resolution. In the present study, the With the sample under a nitrogen purge, the FTIR larger scanner, which can resolve lateral surface spectra were collected using a Mattson Cygnus 100 feature areas as small as 0.05 nm 2, was used to re- spectrometer equipped with a Triglycine Sulfate de- veal the typical features of the porous Si samples tector. Figure 2 shows the FTIR spectra for various and the higher resolution scanner was employed as irradiances during anodization. Peaks are seen at 2225-2050 cm -1, 1250-1000 cm -1, 950-875 cm -1, and a check. The vertical resolution depends on the lo- 66 cm -1. In addition, a shoulder at 1060 cm -1 ap- cal environment of the tip and the morphology of pears for the 8 and 10 mW samples. The peaks from the porous Si samples. The scanner was calibrated 2135 to 2089 cm -~ are generally attributed to the against a Au ruling and the error was less than 10% stretching modes of Sill and SiHz. The peak at 1105 under low thermal drift. All the AFM images re- cm -1 corresponds to the bulk Si-O-Si asymmetric ported here were obtained by the constant deflec- stretching mode and the 907 cm -~ peak is the scis- tion mode, i.e. a feedback loop adjusted the piezo sors mode of Sill2. The Si-O-Si peak is also present scanner so that a constant vertical force was main- in nonporous Si. In addition, there is a Si-Si stretch- tained as the probe scanned across the surface. The ing mode at 617 cm -~ which is obscured by the de- AFM surface tracking ability was also profoundly formation modes of the Si hydrides. 14-15 The inten- affected by the shape of the probe and several can- sity of the SiH-SiHz stretch modes and the Sill2 tilevers were used to assure optimum AFM reso- scissors mode increase with increasing illumination lution. Figure 3 (a), (b) and (c) shows the AFM im- level up to 6 mW. However the intensity of these ages of the surface of porous Si for the 2, 6 and 10 modes for 8 and 10 mW decreases to about the same mW samples, respectively. The lateral feature size strength as that for the 4 mW sample. The Si-Si at the surface of the samples monotonically de- stretching mode also increases up to 6 mW but above creases with increasing illumination level during 6 mW, decreases to a strength lower than that at 2 anodization. We note that characteristic features <10 mW. This indicates that pore surface area increases nm were not observed. Effects of Illumination During Anodization of Porous Silicon 997 < >, 0 mW ..~,_ (n P" mw P, mW (/) (1. X mw (a) mW mW mW 96.0 97.5 99.0 100.5 102.0 103.5 105.0 Si 2p Binding Energy (eV) Fig. 4 -- XPS spectra of Si 2p HV (Si-O) and LV (Si-Si) for po- rous Si. E. X-ray Photoelectron Spectroscopy XPS analysis was performed in a Vacuum Gen- erator ESCALAB MARK I (Mg Ka source, 330 W, (b) 50 eV pass energy).
Details
-
File Typepdf
-
Upload Time-
-
Content LanguagesEnglish
-
Upload UserAnonymous/Not logged-in
-
File Pages6 Page
-
File Size-