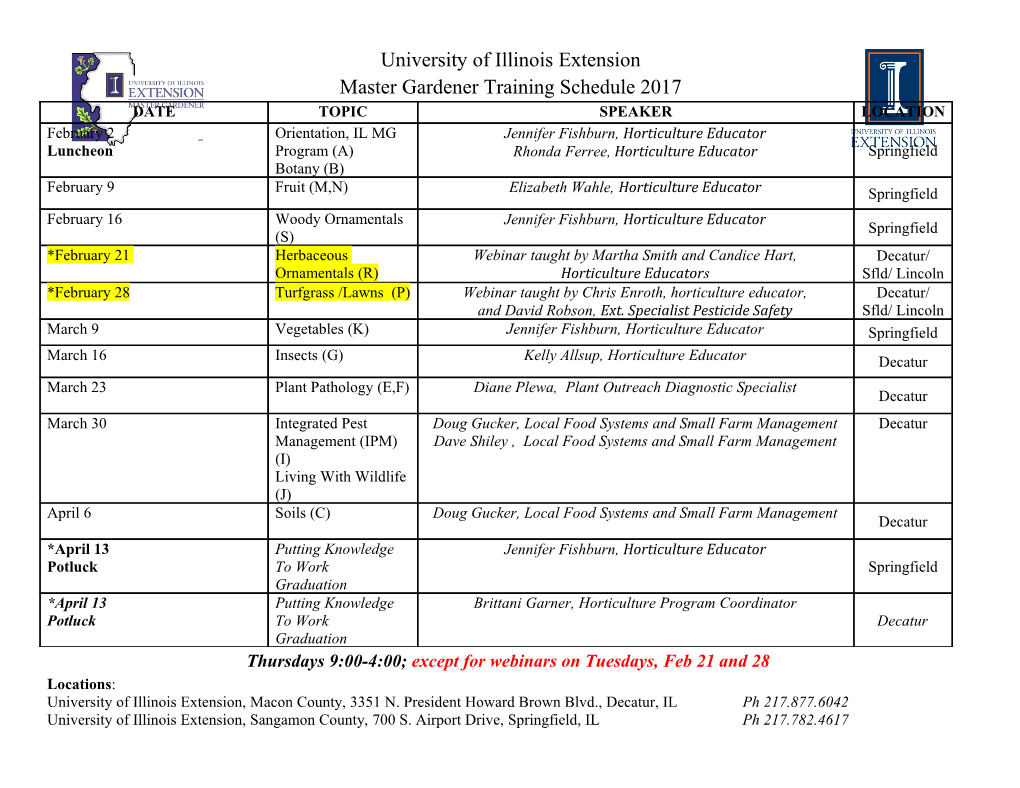
ARTICLES PUBLISHED: 17 JULY 2017 | VOLUME: 2 | ARTICLE NUMBER: 17113 High-concentration planar microtracking photovoltaic system exceeding 30% eciency Jared S. Price1†, Alex J. Grede1†, Baomin Wang1†, Michael V. Lipski1, Brent Fisher2, Kyu-Tae Lee3, Junwen He4, Gregory S. Brulo5, Xiaokun Ma5, Scott Burroughs2, Christopher D. Rahn5, Ralph G. Nuzzo4, John A. Rogers3 and Noel C. Giebink1* Prospects for concentrating photovoltaic (CPV) power are growing as the market increasingly values high power conversion eciency to leverage now-dominant balance of system and soft costs. This trend is particularly acute for rooftop photovoltaic power, where delivering the high eciency of traditional CPV in the form factor of a standard rooftop photovoltaic panel could be transformative. Here, we demonstrate a fully automated planar microtracking CPV system <2 cm thick that operates at fixed tilt with a microscale triple-junction solar cell at >660 concentration ratio over a 140◦ full field of view. In outdoor testing over the course of two sunny days, the system operates× automatically from sunrise to sunset, outperforming a 17%-ecient commercial silicon solar cell by generating >50% more energy per unit area per day in a direct head-to- head competition. These results support the technical feasibility of planar microtracking CPV to deliver a step change in the eciency of rooftop solar panels at a commercially relevant concentration ratio. he economic landscape of photovoltaic (PV) power has on a laterally moving solar cell array have previously shown promise fundamentally changed. Driven by manufacturing advances in this regard but have never been demonstrated at a fully opera- Tand economies of scale over the past decade, silicon and thin- tional level14. film PV module costs have decreased dramatically, far outpacing Here we report a prototype µPT CPV system that operates with a balance of system and soft cost reductions. Toa growing extent, solar triple-junction (3J) microscale photovoltaic (µPV) cell at CR > 660 panels are no longer the dominant cost of the power they produce1–3. over a 140◦ full field of view with peak power conversion efficiency This previously enviable situation has renewed emphasis on exceeding 30%. Implemented with a microcontroller and tested the value of high efficiency since generating more power from a outdoors at latitude tilt in central Pennsylvania, the system generates given system leverages its overall cost. Multijunction concentrating 54% more energy per unit area over an entire day than an adjacent photovoltaics (CPV) have long been pursued as a practical path commercial Si solar cell with 17% power conversion efficiency. to achieve high efficiency, culminating to date in commercial These results represent an important step toward the goal of high- CPV modules that are nearly twice as efficient as silicon and efficiency tracking-integrated CPV in the form factor of a standard thin-film PV panels (CPV power conversion efficiency ηP > 35% rooftop photovoltaic panel. 1,3–5 versus 15 < ηP < 20% typical of PV modules) . Existing CPV systems, however, tend to be large (commercial systems are often High-gain planar microtracking concentration larger than automobiles) and rely on precision, dual-axis rotational Figure 1a illustrates the basic µPT optical system geometry, where a tracking, which is incompatible with deployment on rooftops and in central glass sheet containing a µPV cell slides freely between a pair constrained-space urban environments where a significant fraction of planoconvex refractive and reflective optics, lubricated by index- of worldwide photovoltaic power is generated. matching oil. This catadioptric design folds the optical path and Various tracking-integrated CPV concepts are being explored maintains a flat intermediate focal plane, resolving the usual Petzval to address this challenge, with the ultimate goal of realizing high- curvature problem (where the focal spot degrades with increasing efficiency CPV in the form factor of a standard, fixed-tilt PV incidence angle)6–9 to enable lateral microtracking over a wide range panel6–15. This is particularly demanding from an optical concen- of incidence angles14. A complete panel would consist of top and tration standpoint, since the optical system must be very compact bottom lenslet arrays matched to a corresponding array of µPV cells. (that is, commensurate with typical PV panel thicknesses .2cm) Based on recent work exploring the limits of planar tracking 16 and operate efficiently (optical efficiency, ηopt > 0.8) at high- concentration , this design adopts a low-dispersion N-BK10 glass concentration ratio (commonly defined as CR > 100, where CR is top optic (n ≈ 1.5) in combination with a more dispersive, higher- the ratio of concentrated to incident light intensities) over a wide refractive-index N-LASF31A bottom optic (n ≈ 1.9) that together ◦ range of incidence angles (θ acc = ±70 ) to collect sunlight over help to mitigate chromatic dispersion in a manner analogous to an an entire day. Supplementary Fig. 1 discusses the required angular achromatic doublet. To minimize parasitic reflections, a broadband acceptance. Catadioptric planar microtracking (µPT) systems based fluoropolymer antireflection coating17 is applied to the top lens 1Department of Electrical Engineering, The Pennsylvania State University, University Park, Pennsylvania 16802, USA. 2Semprius Inc., 4915 Prospectus Drive, Suite C, Durham, North Carolina 27713, USA. 3Department of Materials Science and Engineering, University of Illinois at Urbana-Champaign, Urbana, Illinois 61801, USA. 4Department of Chemistry, University of Illinois at Urbana-Champaign, Urbana, Illinois 61801, USA. 5Department of Mechanical and Nuclear Engineering, The Pennsylvania State University, University Park, Pennsylvania 16802, USA. †These authors contributed equally to this work. *e-mail: [email protected] NATURE ENERGY 2, 17113 (2017) | DOI: 10.1038/nenergy.2017.113| www.nature.com/natureenergy 1 © 2017 Macmillan Publishers Limited, part of Springer Nature. All rights reserved. ARTICLES NATURE ENERGY surface and the plano surface of the bottom mirror is coated with a θ = 0° ° inc a 110-nm-thick alumina layer to suppress reflections from the 35 (blue) Lens n = 1.5 to n = 1.9 internal index discontinuity; Supplementary Fig. 2 (green) provides additional details of the optical system. 70° (red) The optical performance of this concentrator is first character- IMF ized in the laboratory under collimated, broadband illumination µ from a supercontinuum laser to mimic low-divergence direct sun- PV light. The top and bottom lens elements are custom-turned aspheres 15 mm and the sliding middle sheet consists of a 600 µm square GaAs µPV cell, transfer-printed and sandwiched between a pair of 0.5-mm- Sliding θ rec thick glass wafers. In this case, the geometric gain (G) given by the glass sheet ratio of the concentrator aperture area (Aconc, defined by the 20 mm lens diameter) to the cell area (Acell), is G = Aconc=Acell = 873. Figure 1b displays the optical efficiency measured as a function Mirror of incidence angle, where ηopt = P cell=P conc is defined as the fraction of optical power incident on the concentrator (P conc) that reaches the b surface of the PV cell (P cell) in the supercontinuum wavelength range 100 GaAs model, G = 873 < λ < absorbed by GaAs (400 873 nm). The concentration ratio 95 follows simply as the product of optical efficiency and geometric 90 gain, CR = Gηopt. A single-junction cell is used in this measurement to avoid errors that might arise from sub-cell current mismatch in 85 GaAs experiment, G = 873 a multijunction PV cell. The results show that ηopt exceeds ∼90% 80 ◦ for incidence angles up to 70 , in agreement with a ray-tracing 75 simulation carried out in Zemax OpticStudio (purple solid line). Based on this validation, the ray-tracing model is subsequently Optical efficiency (%) 70 AM1.5D model, G = 743 extended over a larger wavelength range (350 < λ < 1,850 nm) to 65 predict the optical efficiency for a 650 µm square multijunction cell, 60 which is used in the CPV system detailed below (G = 743, blue 020406080 solid line). The similarity of this result to that for GaAs showcases Angle of incidence (°) the broadband nature of the concentrator, which in turn permits full spectrum concentration for three-, four- or five-junction PV Figure 1 | High-concentration planar microtracking. a, Ray-tracing diagram cells18–20. This remarkable combination of optical efficiency, geo- of the concentrator optical system. The µPV cell is sandwiched between a metric gain, spectral bandwidth and acceptance angle far surpasses pair of glass wafers bonded with optical adhesive and tracking is that of any previous µPT concentrator7,14,15 and even rivals that of accomplished by sliding this composite middle sheet between planoconvex conventional, rotationally tracked CPV concentrators5,21. top and bottom lenses to align with the focal spot at dierent solar incidence angles. The sliding middle sheet is lubricated with CPV system testing index-matching fluid (IMF) to reduce parasitic reflection losses within the The same optics are implemented in a complete, small-scale µPT concentrator stack. b, Optical eciency measured for the concentrator as a CPV system shown in Fig. 2a. The testing jig shown in the photo- function of incidence angle. The red data points are recorded under graph of Fig. 2a is designed to hold the top and bottom lens elements collimated, broadband illumination from a supercontinuum laser using a in place while
Details
-
File Typepdf
-
Upload Time-
-
Content LanguagesEnglish
-
Upload UserAnonymous/Not logged-in
-
File Pages7 Page
-
File Size-