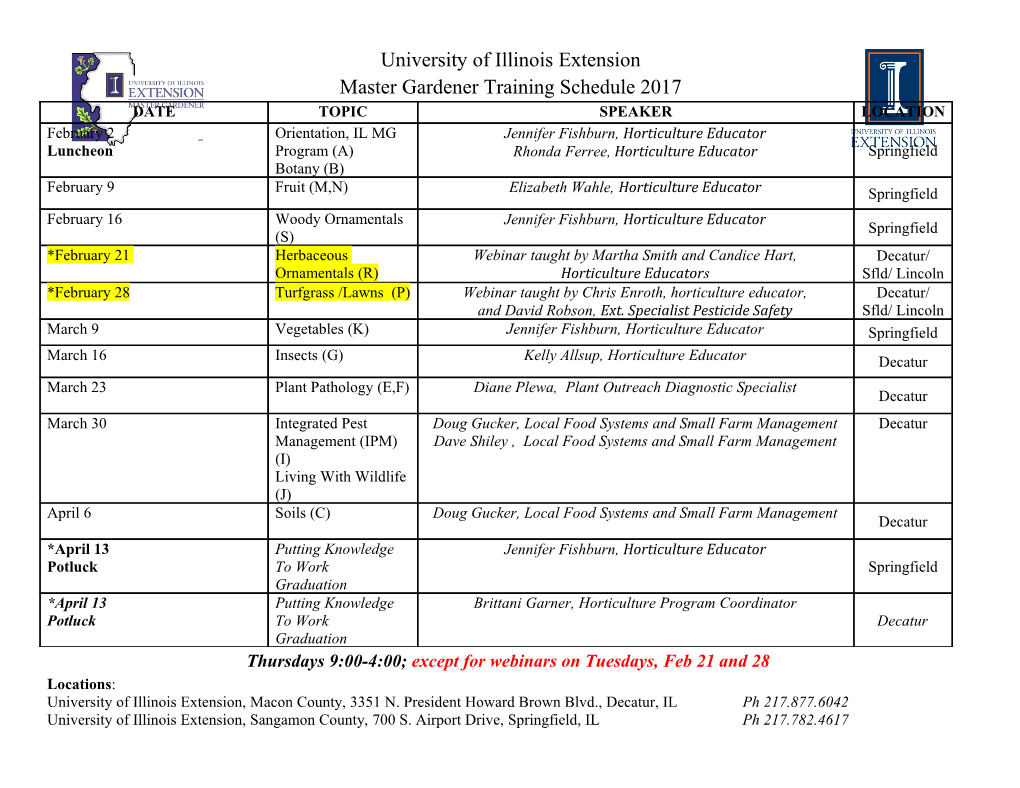
Chapter 8 Application of Spin Labels To Membrane Bioenergetics (Photosynthetic Systems of Higher Plants) Alexander N. Tikhonov1 and Witold K. Subczynski2 1 Department of Biophysics, Faculty of Physics, M. V. Lomonosov Moscow State University, Moscow, 119899, Russia, 2National Biomedical EPR Center, Department of Biophysics, Medical College of Wisconsin, Milwaukee, Wisconsin 53226 Abstract: Following a brief overview of photosynthesis and the macro structure of chloroplasts, examples are presented of the use of nitroxyl spin labels and spin probes to investigate the processes of electron transport, proton transport, and oxygen transfer in chloroplasts. pH-sensitive nitroxyl radicals can be observed inside and outside thylakoids under various conditions, such as different levels of light. Oxygen consumption can be measured via the effect of oxygen on line width of the free radical. Lipid-soluble spin probes monitor changes in the biological membranes. 1. INTRODUCTION Photosynthesis is one of the most important biological processes. The great mass of experimental data obtained in numerous laboratories all over the world has given detailed information on the composition and molecular structure of the light-harvest complexes, electron transport chain (ETC) and ATP synthases in energy-transducing organelles of photosynthetic organisms — higher plants, cyanobacteria and photosynthetic bacteria (Lehninger et al., 1993; Blankenship, 2002). In this chapter, we will consider several aspects of the use of nitroxide radicals to investigate the processes of electron and proton transport, as well as the related processes of oxygen transfer, in chloroplasts — the energy-transducing organelles of the plant cell. Figures 1 and 2 depict a cross section of a chloroplast and a schematic of a chloroplast’s ETC. In higher plants and cyanobacteria, photosynthetic ETC converts the energy of light absorbed by the light-harvesting complexes into the energy of macroergic chemical compounds (NADPH and ATP) by 147 148 ALEXANDER N. TIKHONOV AND WITOLD K. SUBCZYNSKI means of three large pigment-protein complexes located in the thylakoid membranes: photosystem 1 (PS1), photosystem 2 (PS2) and cytochrome complex (bf-complex). PS 1 and PS2 contain reaction centers and respectively) activated by light, and a number of incorporated electron carriers (Jordan et al., 2001; Zouni et al., 2001). Light-induced electron transport from the water splitting complex of PS2 to the terminal electron acceptor of PSl is mediated by the bf-complex and three mobile electron carriers, plastoquinone (Q), plastocyanine (Pc) and ferredoxin (Fd), that provide the electron transfer between PS2, the bf-complex, PS 1 and the ferredoxin-NADP-reductase (FNR) complex. Plastoquinone is an essential component of photosynthetic ETC that couples electron transfer to proton translocation across the thylakoid membrane. The oxidized plastoquinone molecule, Q, binds to PS2 at the acceptor side (bound Q is called a secondary plastoquinone, and then accepts two electrons in tandem from the anion radical of tightly bound primary plastoquinone and two protons from the chloroplast stroma. The reduced plastoquinone molecule (plastoquinol, dissociates from PS2 and migrates inside the thylakoid membrane to the bf-complex. According to Mitchell’s Q-cycle mechanism, is oxidized at the site of the bf-complex close to the thylakoid lumen (internal volume of the thylakoid), Plastocyanine is a mobile electron carrier localized in the lumen that couples the bf-complex to the PS1 reaction center Figure 1. Schematic representation of a chloroplast cutaway. Flattened vesicles symbolize the thylakoids that contain the electron transport chain and ATP synthase complexes. Due to the asymmetrical arrangement of ETC complexes in the thylakoid membrane, the functioning of chloroplast ETC causes translocation of protons into the thylakoid lumen, leading to generation of a transmembrane electrochemical gradient of protons, The transmembrane difference in electrochemical potentials (the so-called “proton potential”) is the driving force for proton translocation through ATP synthase (Mitchell, 1961; APPLICATION OF SPIN LABELS TO MEMBRANES 149 1966; Skulachev, 1988; Haraux and de Kouchkovsky, 1998; McCarty et al., 2000). The two components of the proton potential, (transmembrane electric potential difference) and (transmembrane pH difference), actuate the operation of the ATP synthase machinery. However, the question of how much of the free energy is stored in the form of a pH gradient or electric potential difference still remains (Kaim and Dimroth, 1999; Kramer et al., 1999). Figure 2. Arrangement of chloroplast’s ETC and ATP synthase complexes in a thylakoid membrane. Photosystem 2 (PS2) contains the photoreaction center The photoexcited reaction center donates an electron to the primary acceptor pheophitine (Phe). From the reduced Phe molecule, the electron is transferred to the primary plastoquinone and then to the secondary plastoquinone The doubly-reduced secondary quinon accepts two protons from the stroma, The protonated molecule of the secondary plastoquinol dissociates from PS2, then diffuses to the bf-complex. The cytochrome bf-complex and plastcyanine (Pc) mediate the electron transfer from to photosystem 1 (PS1). Photosystem 1 contains the photoreaction center which donates an electron through the system of electron carriers firmly bound to PS1 to soluble ferredoxin (Fd). The electron is then delivered through the ferredoxin-NADP- reductase complex (FNR) to the terminal electrion acceptor For details of the three- dimensional structures of PS1 and PS2 complexes see Jordan et al., 2001, and Zouni et al., 2001. Translocation of hydrogen ions from the thylakoid lumen (internal part of the thylakoid) to the stroma occurs through the membrane sector of ATP synthase complex). For details of the molecular architecture of the ATP synthase see Abrahams et al., 1994 and Stock et al., 1999. The light-induced acidification of the thylakoid lumen also plays an important role in the feedback control of electron flow in chloroplasts. It has long been known that the rate of plastoquinol oxidation by the bf-complex (the rate-limiting step in the electron flow between two photosystems) strongly depends on the intrathylakoid pH (Rumberg and Siggel, 1969; Tikhonov et al., 1981; Blumenfeld and Tikhonov, 1994; Kramer et al., 1999; Jahns et al., 2002). The rate of plastoquinol oxidation decreases with the 150 ALEXANDER N. TIKHONOV AND WITOLD K. SUBCZYNSKI light-induced acidification of the thylakoid lumen. Therefore, the light- induced decrease in the intrathylakoid pH should cause a slowing of the electron flow from PS2 to PS1. The extent of light-induced acidification of the thylakoid lumen depends on the metabolic state of the chloroplasts. During basal electron transport, i.e., when a supply of substrates for ATP formation is limited (metabolic State 4), the efflux of protons through ATP synmase is small. Therefore, rather strong acidification of the thylakoid lumen at an external pH = 7.5-8.0) occurs in State 4, which leads to the essential retardation of electron flow between two photosystems (Fig. 3, bottom drawing). When ADP and inorganic phosphate are present in excess (Fig. 3, upper drawing, metabolic State 3), the intrathylakoid pH also decreases in the course of chloroplast illumination, but less than in State 4 (due to the efflux of protons from the lumen through ATP synthase in State 3). Since more significant slowing of electron flow occurs at the lower in trathylakoid pH, the rate of electron transport coupled to ATP synthesis (State 3) is supported at a higher level than during the basal electron transport (State 4). Figure 3. Photosynthetic control of electron transport in chloroplasts. Upper drawing depicts a thylakoid functioning under conditions of intensive ATP synthesis (electron transport is coupled to ATP formation, metabolic State 3). Bottom drawing depicts a thylakoid in a state of photosynthetic control (basal electron transport, metabolic State 4). ATP synthase is the reversible molecular machine that can provide ATP from ADP and orthophosphate (State 3) or hydrolyze ATP to ADP and (State 4). In this chapter, we will consider how different kinds of spin labels can be used to investigate the structural and functional characteristics of chloroplasts. Of course, we do not pretend to completely review the great body of literature regarding the numerous aspects of using spin labels in APPLICATION OF SPIN LABELS TO MEMBRANES 151 bioenergetics (see, for instance, Kocherginsky and Swartz, 1999). We will focus our attention on three topics: (1) new applications of pH-sensitive spin labels to measure the proton potential in chloroplasts (Section 2), (2) oximetry of photosynthetic systems (Section 3), and (3) elucidation of the role of the physical state of thylakoid membranes in the regulation of photosynthetic activity of chloroplasts (Section 4). These topics have been at the center of the authors’ interests for the two last decades, and therefore, most of the illustrations presented in this chapter have been redrawn from our previously published papers. 2. THE USE OF PH-SENSITIVE SPIN LABELS TO MEASURE THE PROTON POTENTIAL IN CHLOROPLASTS The question ‘How acidic is the lumen?’ (Kramer et al., 1999) is still debated in bioenergetics literature because the transmembrane pH difference is one of the most important parameters of membrane bioenergetics. In contrast to mitochondria and bacteria that use mostly
Details
-
File Typepdf
-
Upload Time-
-
Content LanguagesEnglish
-
Upload UserAnonymous/Not logged-in
-
File Pages49 Page
-
File Size-