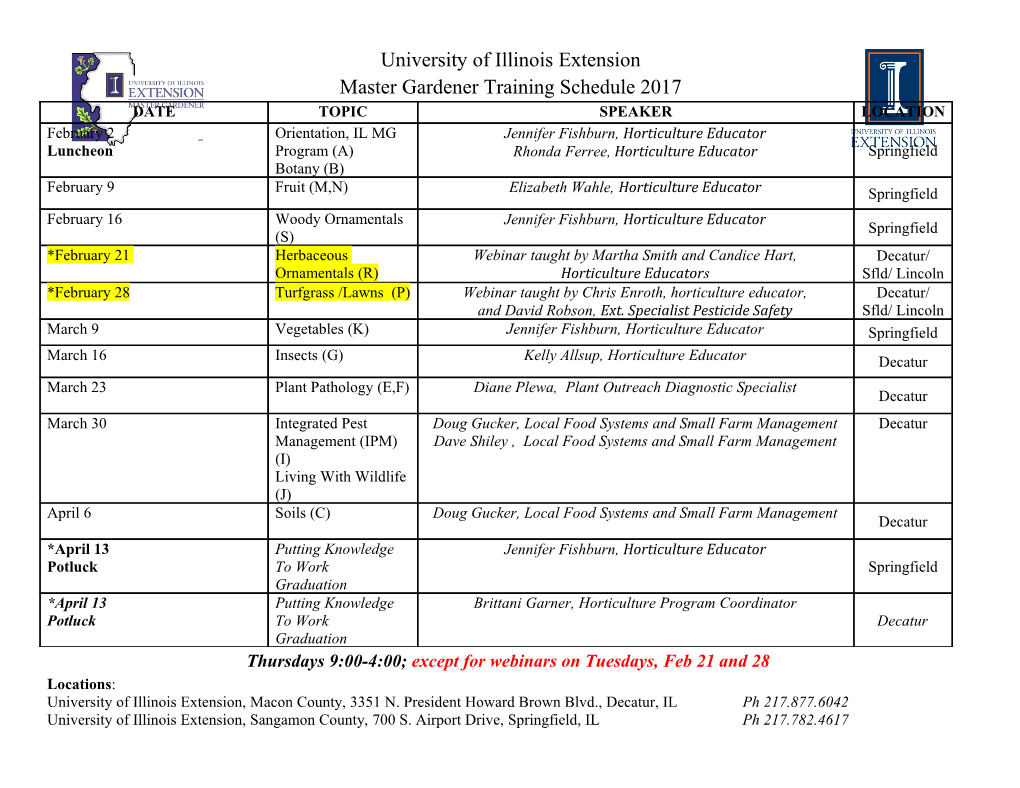
IMPROVING QPE AND VERY SHORT TERM QPF An Initiative for a Community-Wide Integrated Approach BY STEVEN V. VASILOFF, DONG-JUN SEO, KENNETH W. HOWARD, JIAN ZHANG, DAVID H. KITZMILLER, MARY G. MULLUSKY, WITOLD F. KRAJEWSKI, EDWARD A. BRANDES, ROBERT M. RABIN, DANIEL S. BERKOWITZ, HAROLD E. BROOKS, JOHN A. MCGINLEY, ROBERT J. KULIGOWSKI, AND BARBARA G. BROWN A multisensor applications development and evaluation system at the National Severe Storms Laboratory addresses significant gaps in both our knowledge and capabilities for accurate high-resolution precipitation estimates at the national scale. ater is a precious resource and, when ex- collectively as quantitative precipitation information cessive or in short supply, a source of many (QPI).] To meet these needs at the national scale, W hazards. It is essential to monitor and pre- accurate QPI is needed at various temporal and spatial dict water-related hazards, such as floods, droughts, scales for the entire United States, its territories, and debris flows, and water quality, and to determine immediate surrounding areas. Temporal scales current and future availability of water resources. range from minutes to several hours for flash flood Accurate quantitative precipitation estimates (QPE) prediction. QPI products can then be aggregated to and very short term quantitative precipitation fore- support longer-term applications for water supply casts (VSTQPF) provide key input to these assess- prediction. Spatial scales range from a few square ments. [QPE and VSTQPF are hereafter referred to kilometers or less for urban flash flood prediction, AFFILIATIONS: VASILOFF, HOWARD, RABIN, AND BROOKS—NOAA/ MCGINLEY—NOAA/Earth System Research Laboratory, Boulder, National Severe Storms Laboratory, Norman, Oklahoma; SEO— Colorado; KULIGOWSKI—NOAA/National Environmental Satellite, NOAA/NWS/Office of Hydrologic Development, Silver Spring, Data, and Information Service, Camp Springs, Maryland Maryland, and University Corporation for Atmospheric Research, CORRESPONDING AUTHOR: Steven Vasiloff, NOAA/National Boulder, Colorado; ZHANG—Cooperative Institute for Mesoscale Me- Severe Storms Laboratory, National Weather Center, 120 David L. teorological Studies, University of Oklahoma, and NOAA/OAR Na- Boren Blvd., Norman, OK 73072 tional Severe Storms Laboratory, Norman, Oklahoma; KITZMILLER— E-mail: [email protected] NOAA/NWS/Office of Hydrologic Development, Silver Spring, The abstract for this article can be found in this issue, following the table Maryland; MULLUSKY—NOAA/NWS/Office of Climate, Water, and of contents. Weather Services, Silver Spring, Maryland; KRAJEWSKI—IIHR—The DOI:10.1175/BAMS-88-12-1899 University of Iowa, Iowa City, Iowa; BRANDES AND BROWN—National Center for Atmospheric Research, Boulder, Colorado; BERKOWITZ— In final form 15 June 2007 NOAA/WSR-88D Radar Operations Center, Norman, Oklahoma; ©2007 American Meteorological Society AMERICAN METEOROLOGICAL SOCIETY DECEMBER 2007 | 1899 to several thousand square kilometers or more for benchmarking are often lacking. A case in point is climate prediction. Spurred by advances in weather that issues identified as being the most important for radars, satellites, and numerical weather prediction radar rainfall estimation over a quarter of a century (NWP), the science and operational practices of pre- ago (Wilson and Brandes 1979) are still identified as cipitation analysis and prediction have, for the most the most important today. More importantly, it is just part, made tremendous progress in the last quarter as difficult today, as it was then, to ascertain the best century. However, as described below, significant gaps available methodology, technique, or algorithm for a in both knowledge and capabilities must be bridged to particular problem (e.g., rain gauge–based correction produce accurate high-resolution QPI at the national of bias in radar rainfall data). To effectively address scale for a wide spectrum of users. In this article, we the nation’s needs, QPI must be recognized as a mul- present a proposal for a community-wide integrated tifaceted, multiscale, multisensor (MS) problem that approach that we argue is necessary to advance pre- requires concerted and coordinated efforts that are cipitation science and its infusion into operations to community wide and integrated. A community-wide meet the nation’s QPI needs effectively. approach is necessary because the problem is large and The time scale of focus for next-generation QPI, complex and, hence, requires the expertise and experi- or Q2, products is about a day into the past and ences of many. An integrated approach is necessary to about 6 hours into the future (Fig. 1). Going back fully capitalize on advances in science and technology in time provides an opportunity for near-real-time in many different areas. precipitation reanalysis, or analysis of record, that To foster the development of a community-wide may fully utilize observations that are not available integrated approach of advancing QPI science and for real-time estimation. Looking into the very short its infusion into operations, the National Oceanic term future provides an opportunity to combine QPE and Atmospheric and Administration (NOAA)/ with an analysis of the current state of the atmosphere National Severe Storms Laboratory (NSSL) and the and NWP output to produce seamless precipitation NOAA/National Weather Service (NWS)/Office of forecasts at the local to continental spatial scale. Hydrologic Development (OHD) jointly organized Compared to numerical modeling for weather or and hosted the first Q2 workshop in Norman, hydrologic prediction, QPI has remained a loosely Oklahoma, on 28–30 June 2005 (proceedings avail- organized area of research. As such, algorithms and able online at www.nssl.noaa.gov/projects/q2/ applications have often been developed by different 2005wkshp/). This article synthesizes the workshop people in different organizations in different places discussions and outlines a comprehensive Q2 science (often from scratch) for specific users and applica- plan. A suggested path for research to operations tions. While this mode of discovery has resulted in (RTO) is also provided with the caveat that any RTO important scientific advances and valuable operational plan must follow the NWS Operations and Services experiences, these advancements are less likely to reach Improvement Process (OSIP; NWS 2007). As seen the entire research and operational community. Even below, Q2 shares a number of focus areas and strate- if they do, meaningful comparative verification and gies for research and development (R&D) and RTO transition with warm- and cool-sea- son QPF improvement (Fritsch and Carbone 2004; Ralph et al. 2005). As such, close and cross-cutting collaborations with the QPF com- munity, as well as the hydrology and water resources communities, are critical to its success. Data sources for quantitative precipitation information. A large part of the workshop was devoted to critically reviewing currently FIG. 1. Schematic depiction of the time scale of focus for next- available observational tools for generation quantitative precipitation information (Q2). “Seamless” QPI, operational practices and ex- means that discontinuities between observations and model data periences, key science issues, and have been removed by the blending processes. future prospects for technological 1900 | DECEMBER 2007 advances with respect to each tool. This section sum- the radar beam is close to the ground. In the current marizes those discussions that provide the basis for WSR-88D network configuration, coverage of storms Q2. (Details can be found in the individual presen- above 1 km is especially poor in the West (Maddox tations available online at http://nssl.noaa.gov/proj- et al. 2002), where the NWS River Forecast Centers ects/q2/2005wkshp/presentations.php.) Table 1 lists (RFCs) use primarily rain gauge data to estimate the primary tools for estimating precipitation, along precipitation input to operational hydrologic models. with the temporal and spatial scales of observations There has been an increasing demand for scanning and their current operational applications. Strategies strategies with lower starting elevation angles for for combining data from the three primary sensors mountain-top radar locations and for better detection are discussed in more detail later. of shallow stratiform precipitation (e.g., Wood et al. 2003); these radars do not scan below 0.5°. In addition Weather radar. The major remote sensing tool for to modified scanning strategies, gaps in WSR-88D precipitation over the United States is the Weather coverage may be filled by other radars such as those Surveillance Radar-1988 Doppler (WSR-88D; Fulton used by the Federal Aviation Administration (Vasiloff et al. 1998). Key geometric uncertainties in radar esti- 2001), and by limited-range radars such as those in mates are due to the curvature of the Earth and radar the planned Collaborative Adaptive Sensing of the beam broadening (e.g., Battan 1973). Precipitation Atmosphere radar network (McLaughlin et al. 2005). estimates are expected to be most accurate where Television station radars also could be adapted into TABLE 1. The three primary sensors used to estimate precipitation, their associated strengths and weak- nesses, time and spatial scales of measurements, and current operational applications. Time–space Sensor Strengths Weaknesses Operational applications scales Weather radar • High spatial and • Range effects • 5–10
Details
-
File Typepdf
-
Upload Time-
-
Content LanguagesEnglish
-
Upload UserAnonymous/Not logged-in
-
File Pages13 Page
-
File Size-