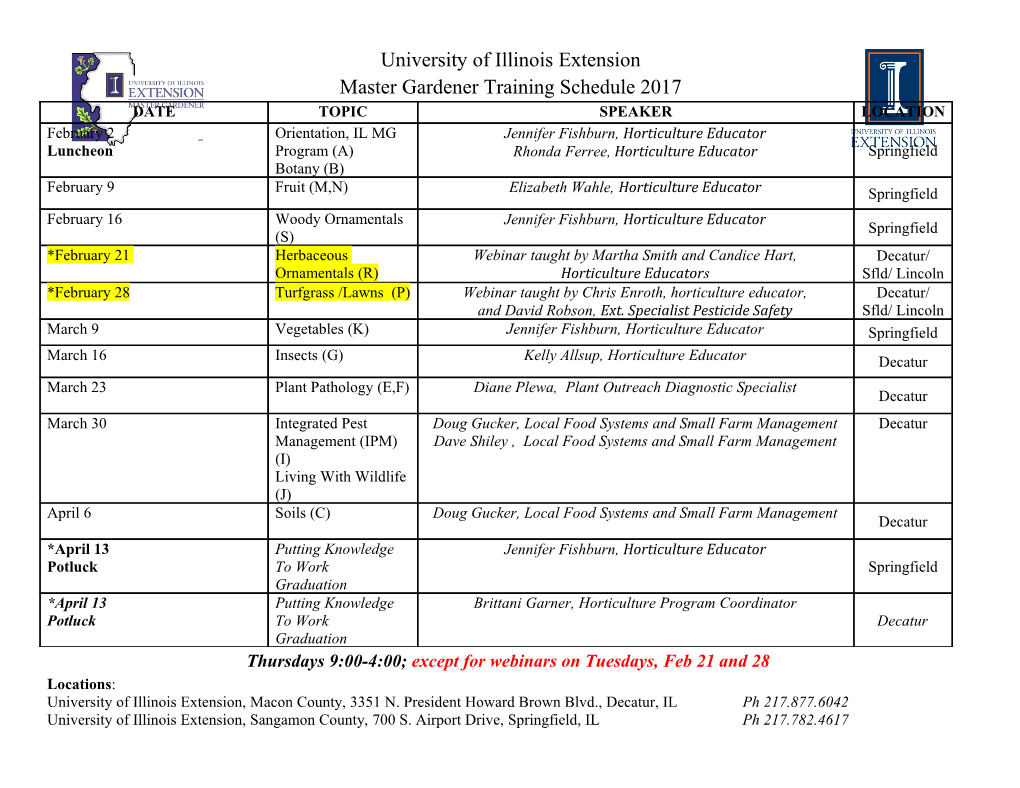
Nanophotonics 2021; 10(9): 2389–2406 Review Felix Sima* and Koji Sugioka* Ultrafast laser manufacturing of nanofluidic systems https://doi.org/10.1515/nanoph-2021-0159 Keywords: glass; lab-on-chip; nanofluidics; polymers; ul- Received April 12, 2021; accepted May 25, 2021; trafast lasers. published online June 11, 2021 Abstract: In the last decades, research and development 1 Introduction – from micro- to of microfluidics have made extraordinary progress, since they have revolutionized the biological and chemical fields nanofluidics as a backbone of lab-on-a-chip systems. Further advance- ment pushes to miniaturize the architectures to nanoscale Microfluidics is the field that has been dedicated to the in terms of both the sizes and the fluid dynamics for some miniaturization and fluidic manipulation at microscale specific applications including investigation of biological addressing integration of biological tools and functions in sub-cellular aspects and chemical analysis with much order to save systematic labor, large space, working times, improved detection limits. In particular, nano-scale chan- and expensive costs [1, 2]. By developing complex micro- nels offer new opportunities for tests at single cell or fluidic systems and control fluid dynamics by automati- even molecular levels. Thus, nanofluidics, which is a zation, robotic workstations offer nowadays possibilities to microfluidic system involving channels with nanometer solve essential issues for chemistry, biology and medicine. dimensions typically smaller than several hundred nm, has Lab-on-chip (LOC) devices and micro-total analysis been proposed as an ideal platform for investigating systems (μTAS) have been then proposed to tackle specific fundamental molecular events at the cell-extracellular applications, which typically involve microfluidics to be milieu interface, biological sensing, and more recently for used for separation techniques [3–8], micro-electro studying cancer cell migration in a space much narrower mechanical systems (MEMSs) [9–12], clinical applications than the cell size. In addition, nanofluidics can be used for [13–15], forensic or molecular diagnostics [16–18], and sample manipulation in analytical chemistry, such as proteomics [19–21] or for innovative tools enabling sample injections, separation, purifications or for quanti- advanced research in the cancer field [22–26]. Fluid tative and qualitative determinations. Among the nano- dynamics associated with microfluidics is dominated by fabrication technologies, ultrafast laser manufacturing is a inertial nonlinearity while the mass transport in such promising tool for fabrication of nanofluidics due to its devices is controlled by viscous dissipation [27]. Specif- flexibility, versatility, high fabrication resolution and three ically, viscous dissipation of mechanical energy induces dimensional (3D) fabrication capability. In this paper, we fluidic resistance and transformation into heat due to review the technological advancements of nanofluidic internal friction [28]. Reynolds [29] and Peclet [30] are two systems, with emphasis on fabrication methods, in dimensionless numbers often used in microfluidics to particular ultrafast laser manufacturing. We present the describe the physical phenomena within the microfluidic challenges for issues concerning channel sizes and fluid space. The Reynolds number, Re, measures the ratio dynamics, and introduce the applications in physics, between inertial and viscous force (Eq. 1), while Peclet biology, chemistry and engineering with future prospects. number, Pe, defines the importance of convection in respect to diffusion (Eq. 2). Re could be related with Pe by Eq. (3). *Corresponding authors: Felix Sima, CETAL, National Institute for ρ v l R = Laser Plasma and Radiation Physics, Atomistilor 409, 077125, e μ (1) Magurele, Romania; and Koji Sugioka, RIKEN Center for Advanced Photonics, Wako, Saitama, Japan, E-mail: felix.sima@inflpr.ro v l P = (F. Sima), [email protected] (K. Sugioka). https://orcid.org/0000- e D (2) 0001-5911-0288 (F. Sima) Open Access. © 2021 Felix Sima and Koji Sugioka, published by De Gruyter. This work is licensed under the Creative Commons Attribution 4.0 International License. 2390 F. Sima and K. Sugioka: Ultrafast laser manufacturing of nanofluidic systems Pe D with living mammalian cells as it offers good permeability Re = (3) ʋ to gases. Exploratory research in microfluidic systems has been carried out using poly(dimethylsiloxane), or PDMS, where ρ is the fluid density, v is the average velocity, µ is the an optically transparent, soft elastomer. dynamic viscosity, l is the characteristic length, ʋ is As the microfluidics field advanced rapidly, it was vorticity difussivity and D is the diffusion coefficient. In further expected to downsize the scale to nanosizes for microfluidic channels, since the flow velocity is low, the Re challenging the physics of fluid flow in ultrasmall becomes small. This reflectsthedominantroleoftheviscosity channels and the possibility of single molecule analysis. over inertial forces that implies the absence of eventual Thus, a new field of nanofluidics has emerged and vortices or turbulences. Then, at such a low Re number, the received considerable attention, especially oriented Newton’ssecondlawforfluid particles (known as Navier– towardsapplicationsofsmallsystemsthatinclude Stokes flow state equation) which governs the fluid motion microarrays for DNA sequencing, microfluidic devices can be estimated as [31]: for polymerase chain reaction (PCR), LOC systems for μ∇2v = ∇p (4) synthesis and analysis of peptide and oligonucleotide libraries, microchips for drug screening or for single where p is the fluid pressure. It evidences that the fluid cell analysis. Nanofluidics can be also useful for sample velocity is given by pressure distribution only, the inertial manipulations in analytical chemistry, such as sample force is negligible, the diffusion is dominant while the injections, separation, purifications for quantitative and motion could be considered symmetric in time [32]. qualitative analysis. However, different derived flow states can be applied for The nanofluidics was defined as the study of fluid topologically optimized configurations of microfluidic transport in or around nanosized objects or, more devices designed for specific applications [33]. concretely, the microfluidic system with at least one Early works in fluidic microsystems used either silicon characteristic dimension below 100 nm [37, 38] while or glass as platform materials. In particular, micro- extended nanofluidics is considered to include the engi- fabrication based on photolithographic techniques was neering of fluids in channels of submicrometer dimensions applied to single crystalline silicon or glass for obtaining [39]. A channel surface usually gets electrostatic charges in structures for flow injection analysis, electrophoresis and contact with aqueous solutions. It then binds counter-ions detection [4, 34]. Compared to glass, silicon is rather from liquid, developing an electric double layer (EDL) close expensive and not suitable for the analysis by conventional to the surface. The excess counter-ions will travel, inducing optical methods due to opaque characteristics in a visible liquid motion by viscosity consequence. This motion is range. These materials have been recently replaced by known as electroosmotic flow [40]. The characteristic polymers which contribute to rapid advancement of the thickness of the EDL, known as Debye length, is given by microfluidics field. Indeed, the introduction of soft lithography for polymer microfabrication had a huge 1 λD = , (5) impact on microfluidics as it was a simple technique k enabling fabrication of micro-systems without the need of where k is a constant related to the ionic composition and using clean room equipment. Soft lithography employs defines the equilibrium distribution of ions diffused in the non-photolithographic strategy for micro- and nano- the EDL. In contrast to the microscale channels where the fabrication. By using an elastomeric stamp with relief thickness of the EDL can be completely negligible, this structures one may create patterns with micro or even nano becomes critical for the flow in nanochannels in which its scale feature sizes down to 30 nm [35]. These characteris- dimensions may be comparable or even smaller than the tics were then exploited to control patterning of complex Debye length. Thus, the mass transport in nanofluidic molecules relevant to biology or to fabricate channel net- systemsisdifferentascomparedwiththatinmicrofluidic works appropriate for use in microfluidics for cellular systems since the electric potential barrier given by a manipulation. The soft lithography is also the convenient, large EDL affects transport properties of ionic species. inexpensive, and rapid technology to produce configura- However,formostmicrofluidic applications, the EDL tions of large feature sizes used in biology (≥50 µm) without thickness may be negligible in the study of electroos- considerable effort [36]. In addition, as compared with rigid motic flow, if λD/h ≪ 1, where h is channel height. materials, the elastomers offer a simpler alternative to Nevertheless, the Navier–Stokes flow state equation fabricate components required for microanalytical systems (Eq. 4) was also accepted to the nanoscale while specific such as pumps and valves, and are also adequate to work transport phenomena appears due to electrostatics and F. Sima and K. Sugioka: Ultrafast laser manufacturing of nanofluidic
Details
-
File Typepdf
-
Upload Time-
-
Content LanguagesEnglish
-
Upload UserAnonymous/Not logged-in
-
File Pages18 Page
-
File Size-