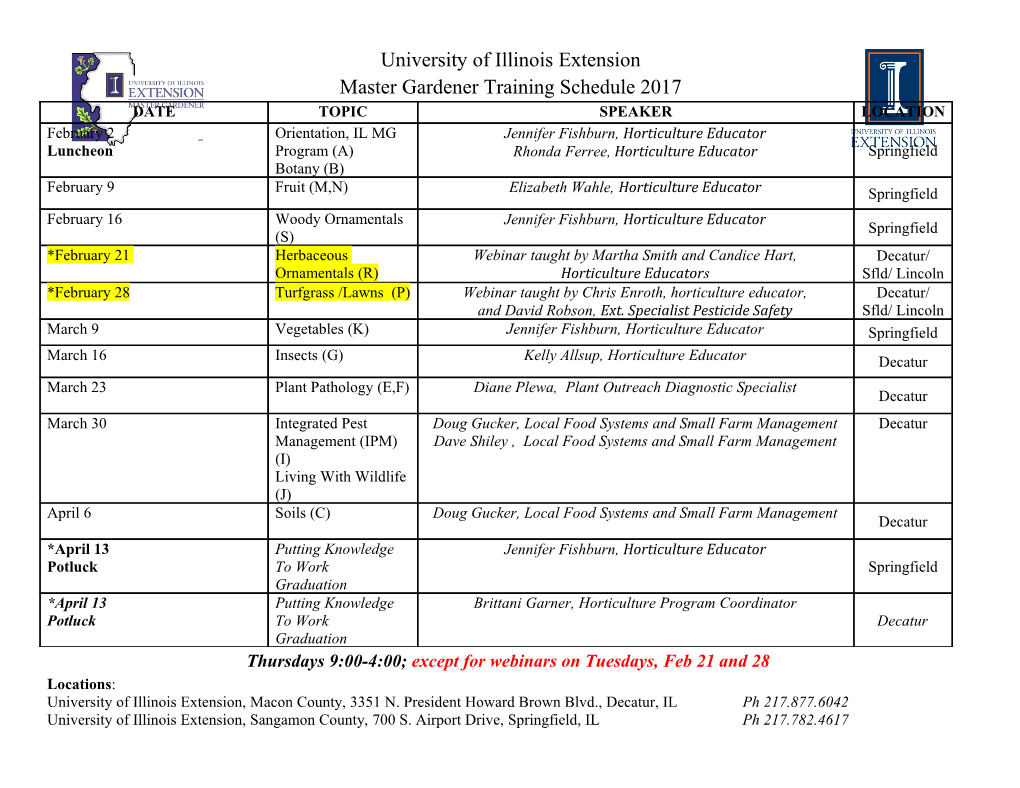
C. E. Torres-Cerna et al., Batch Cultivation Model for Biopolymer Production, Chem. Biochem. Eng. Q., 31 (1) 89–99 (2017) 89 Batch Cultivation Model for Biopolymer Production This work is licensed under a a,◊ a,◊,* b Creative Commons Attribution 4.0 C. E. Torres-Cerna, A. Y. Alanis, I. Poblete-Castro, International License and E. A. Hernandez-Vargasc,d,* aCUCEI, Universidad de Guadalajara, Blvd. Marcelino Garcia Barragan 1421, Col. Olimpica, Guadalajara, Jalisco, México, C. P. 44430 bUniversidad Andrés Bello, Faculty of Biological Sciences, Center for Bioinformatics and Integrative Biology, Biosystems Engineering Laboratory, 8340176 Santiago, Chile cSystems Medicine of Infectious Diseases, Helmholtz Centre doi: 10.15255/CABEQ.2016.952 for Infection Research, Inhoffenstr. 7, 38124 Braunschweig, Germany Original scientific paper dFrankfurt Institute for Advanced Studies (FIAS), Received: July 19, 2016 Ruth-Moufang-Str. 1, 60438 Frankfurt am Main, Germany Accepted: March 7, 2017 This paper presents a mathematical model to evaluate the kinetics of two different Pseudomonas putida strains, wild and mutant-type for the microbial production of poly- hydroxyalkanoates (PHAs). Model parameters were estimated to represent adequately experimental data from the batch reactor using the differential evolution algorithm. Based on the mathematical model with the best-fit parameter values, simulations suggested that the high production of PHA by the mutant strain can be attributed not only to the higher production of PHA but also to a reduction in the consumption rate of the substrates of approximately 66 %. Remarkably, the cell growth rate value is higher for the wild type than the mutant type, suggesting that the PHA increase is not only to an increase in the production rate but also to the metabolism of the cells. This mathematical model advanc- es comprehension of the PHA production capacity by P. putida paving the road towards environmentally friendly plastics. Key words: mathematical modeling, batch cultivation kinetics, biopolymers, Pseudomonas putida, polyhydroxyalkanoates Introduction called bioplastic materials. The replacement of a fraction of synthetic plastics with biodegradable Plastics are one of the most used materials polymers produced from renewable resources offers worldwide and their production rate is increasing to solve important problems such as the overall over the years. In 2013, the worldwide plastic mate- consumption of fossil fuels, environmental pollu- rial production was approximately 299 million tons, tion, and solid waste management3. Bioplastics can and these numbers continue to increase, causing easily be degraded (Figure 1), and in this way, the large amounts of waste which highly contributes to plastics deposited in nature could be largely de- 1 the pollution of the environment . Plastic materials creased, serving as a carbon substrate for different have been for a long time produced through petro- microorganisms. chemicals routes. Theses plastics have a high dura- Bioplastics are available in specific areas and bility and are resistant to degradation. Although applications, for example composting bags and such properties are considered important for the in- sacks, fast food tableware (cups, plastic bottles, cut- dustrial production of plastics, these are a signifi- lery, etc.), agriculture and hygiene4. Furthermore, cant source of environmental and waste manage- ment problems2. biopolymers are also used in most industries for technical applications, including medicine and phar- New avenues of research have been developed macy. Among the candidates for biodegradable to produce plastics with biodegradability properties, plastics, polyhydroxyalkanoates (PHAs) have been drawing much attention because of their similar ma- *Corresponding authors: E-mail: [email protected] (EHV) terial properties to conventional plastics and com- [email protected] (AYA) plete biodegradability. PHAs are insoluble in water ◊These authors contributed equally to this work. and exhibit a rather high degree of polymerization C. E. Torres-Cerna et al., Batch Cultivation Model for Biopolymer Production 89–99 90 C. E. Torres-Cerna et al., Batch Cultivation Model for Biopolymer Production, Chem. Biochem. Eng. Q., 31 (1) 89–99 (2017) 13 Production P. putida Microorganism( ) Cycleofbioplastics in nature Plasticproduct Compost(as food formicroorganisms) Wastefor disposal Fig. 1 – Bioplastics cycle. Bioplastics are produced by microorganisms, which are collected after being Fig. 1 Bioplastics cycle. Bioplastics usedare pr asodu solidced by wastes. microorganisms These are, wh degradedich are colle andcted used after asbein carbong used assources solid wa forstes the. Th microorganisms.ese are degraded and used as carbon sources for the microorganisms. ranging from 105 to 107 Dalton (Da). Their non-tox- tween petroleum and bacterial-based production of icity and biodegradability properties are essential polymers. For example, the in silico driven meta- 3 Newfor theiravenues potential of research use . have been developed to producebolic plasticsengineering with approachbiodegradability developed by Po- PHAs are naturally produced as granules in the blete-Castro and coworkers, re-designed P. putida properties,cytoplasm called of cells biop bylastic numerous materials. bacteria The under replacement lim- to of enhance a fraction the productionof synthetic of mcl-PHAsplastics using glu- iting nutrients and excess carbon source3,5. Pseudo- cose as the carbon source. The metabolically engi- neered strains that were performed predicted only with monasbiode gradableputida strains polymers are the produced most used from bacteria renewable for resources offers to solve important the synthesis of medium-chain-length PHAs (mcl- one key mutation, revealed a 100 % increase in the PHAs) in the presence of different carbon sources, final PHA titer, a 50 % increase in the cellular PHA problemssuch assuch fatty as acids, the overall glucose, consumption and glycerol. ofParticular fossil fuels,content, environmental and 80 % pollution in the PHA, and yield, solid relative to its parent strain. properties of mcl-PHAs3 give them a broader appli- wastecation management range over. short-chain-lengthBioplastics can PHAseasily6 . beUsual degraded- (FAdditionallyigure 1), and to thein thisimprovements way, the through ge- ly, the production process is composed of two netic modifications, mathematical models have plasticsphases. deposited In the firstin natu phase,re couldcells arebe challengedlargely decreased, by proved serving to beas a arelevant carbon tool substrate to enhance for biopolymers growth in a minimal medium with an excess of car- production8. On the one hand, metabolic approaches differentbon microorganisms.source and a limited amount of ammonium as are focused on metabolic pathways for PHA pro- the nitrogen source. The cells rapidly consume the duction, these can reflect the intracellular dynamics. Bioplasticscarbon substrate are avai andla exhibitble in specificexponential areas growth and applications,as There isfor a particularexample interestcomposting for this bags level of model- long as ammonium is present in the medium3,6. The ling due to the high detail that can describe8. Never- second phase is characterized by the transition from theless, this detail level promotes highly4 complex and sacks,a feast fastto a food famine tableware period, which(cups, allows plastic the bottles, high cutlery,models. etc.), On agriculture the other hand, and hygienemodelling. approaches synthesis of mcl-PHA in batch reactors3,6,7. based on the population growth can help dissect the Nevertheless, the amount of bacterial produc- dynamics of different strains8. This approach could tion of mcl-PHA is insufficient compared to the de- be useful in developing different cultivation strate- mand and the reduced quantity of production, influ- gies in order to enhance the PHAs production with- encing the price of bioplastics in the market. out increasing the model complexity9,10. Systems metabolic engineering approaches of Pseu- Recently, several mathematical models, based domonas putida (P. putida) have enhanced the pro- on population growth, have been proposed138 to facil- duction of PHA, thus enabling development of a itate the dynamics of biopolymer production9–14. more efficient strain, which closes the cost gap be- Most of these works contain many parameters esti- C. E. Torres-Cerna et al., Batch Cultivation Model for Biopolymer Production, Chem. Biochem. Eng. Q., 31 (1) 89–99 (2017) 91 mated based on local optimization algorithms. Note where S is the glucose (carbon source), N is the NH4 that parameter estimation is a complex problem in- concentration (nitrogen source), X is the active bio- volving highly non-linear equations and several mass, and P is the biopolymer PHA. NH4 is as- variables. Therefore, global optimization algorithms sumed as the limiting nutrient13 and the total biomass are needed to avoid incorrect fits and consequently (Cell Dry Mass) is composed by the catalytically incorrect interpretations. active biomass and the PHA, thus CDW = X + P. The mechanisms of self-adaption, self-organiz- Consumption rates of substrates by active bio- ing and self-learning in stochastic optimization ap- mass are presented in equations (5) and (6). m and proaches allow addressing challenging problems S m can be interpreted as the specific consumption that cannot be solved by traditional methods. In this N rates of glucose and NH by the active
Details
-
File Typepdf
-
Upload Time-
-
Content LanguagesEnglish
-
Upload UserAnonymous/Not logged-in
-
File Pages11 Page
-
File Size-