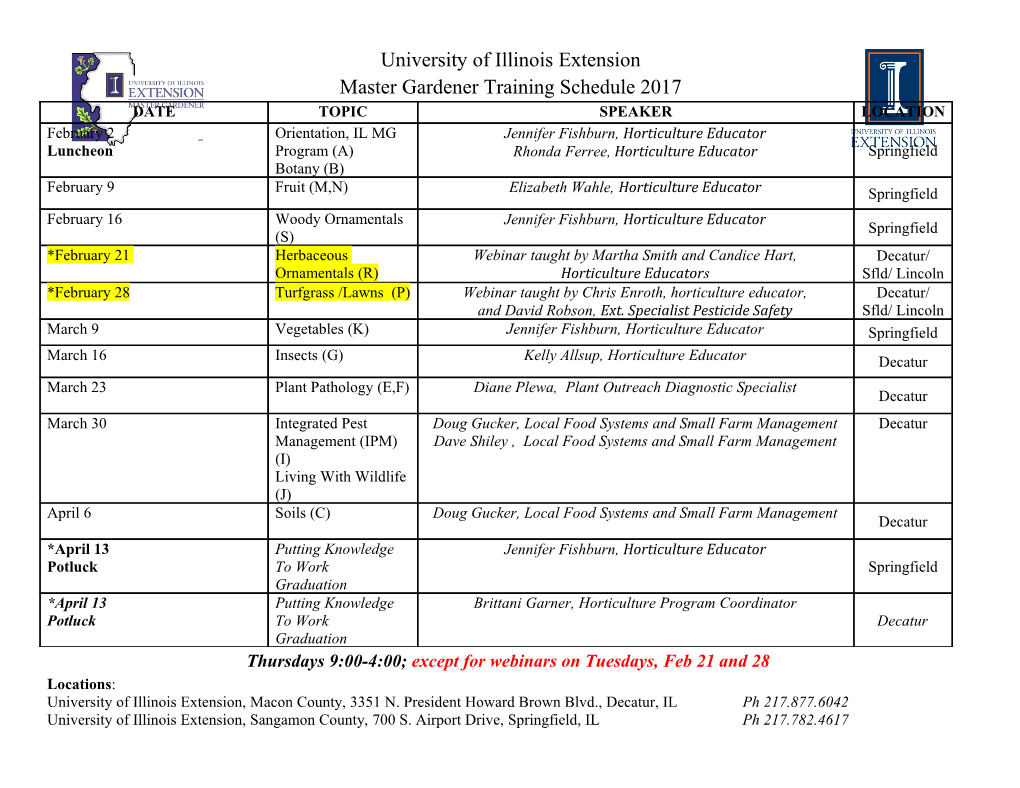
Journal of Geophysical Research: Oceans RESEARCH ARTICLE Topographic Rossby Waves in the Arctic Ocean’s Beaufort Gyre 10.1029/2018JC014233 Bowen Zhao1 and Mary-Louise Timmermans1 Special Section: Forum for Arctic Modeling 1Department of Geology & Geophysics, Yale University, New Haven, CT, USA and Observational Synthesis (FAMOS) 2: Beaufort Gyre phenomenon Abstract A 5-year long time series of temperature and horizontal velocity in the Arctic Ocean’s Key Points: Beaufort Gyre is analyzed with the aim of understanding the mechanism driving the observed variability • Beaufort Gyre mooring on timescales of tens of days (i.e., subinertial). We employ a coherency/phase analysis on the temperature measurements of velocity and and horizontal velocity signals, which indicates that subinertial temperature variations arise from vertical temperature are analyzed for subinertial signal excursions of the water column that are driven by horizontal motions across the sloping seafloor. The • Observations are consistent vertical displacements of the water column (recorded by the temperature signal) show a bottom-intensified with topographic Rossby waves signature (i.e., decay toward the surface), while horizontal velocity anomalies are approximately barotropic propagating on sloping seafloor • Topographic Rossby waves play a role below the main halocline. We show that the different characteristics in vertical and horizontal velocities are in Beaufort Gyre stabilization consistent with topographic Rossby wave theory in the limit of weak vertical decay. In essence, a linearly decaying vertical velocity profile implies that the whole water column is stretched/squashed uniformly with Correspondence to: depth when water moves horizontally across the bottom slope. Thus, for the uniform stratification of the B. Zhao, deep water column, the response in the relative vorticity field (ensuring conservation of potential vorticity) [email protected] is also uniform with depth, leading to the observed barotropic horizontal velocity changes. The prevalent topographic Rossby wave activity is discussed in context with Beaufort Gyre spin-up, dissipation, and Citation: stabilization. Zhao, B., & Timmermans, M.-L. (2018). Topographic Rossby waves in the Plain Language Summary A 5-year long time series of temperature and velocity in the Arctic Arctic Ocean’s Beaufort Gyre. Journal Ocean’s Beaufort Gyre is analyzed to understand the source of water motions that occur with periods of tens of Geophysical Research: Oceans. 123. https://doi.org/10.1029/2018JC014233 of days. We find that vertical water motions are driven by horizontal flows across the sloping seafloor. The vertical motions are strongest near the seafloor. On the other hand, horizontal motions in the deep Beaufort Gyre are approximately uniform with depth. We show that these characteristics are consistent with Received 1 JUN 2018 Accepted 24 AUG 2018 topographic Rossby waves, which are waves associated with water moving horizontally across a seafloor Accepted article online 29 AUG 2018 slope. The prevalent topographic Rossby wave activity is discussed in context with energetics and stabilization of the changing Beaufort Gyre. 1. Introduction The anticyclonic Beaufort Gyre, centered over the Arctic’s Canada Basin, is a dominant feature of the Arctic Ocean circulation. The intensity and energetics of the Beaufort Gyre govern heat and freshwater storage and transport, influencing sea ice and Arctic and global climate (e.g., Proshutinsky et al., 2009). The response of the gyre to changes in wind forcing involves the excitation of Rossby waves; although the polar regions have only weak effect (and hence weak planetary Rossby waves), seafloor topography in the Canada Basin gives rise to topographic Rossby waves (TRWs; e.g., Luneva et al., 2012; Timmermans et al., 2010; Yang et al., 2016). As such, TRWs are an essential element of Beaufort Gyre spin-up, geostrophic adjustment, and dissipation, although they have been little studied in observations. This study analyzes Beaufort Gyre mooring measurements to understand the presence and dynamics of TRWs. The Beaufort Gyre is characterized by a strong halocline stratification and, below around 300-m depth, a deeper more weakly stratified ocean (Figures 1 and 2). The temperature stratification is dominated by the warm core of the Atlantic Water Layer centered around 450-m depth (Figure 1). Deeper than this, there are subtle yet rather complex water mass layers, the evolution of which have been examined in a number of stud- ies (e.g., Carmack et al., 2012; Dosser & Timmermans, 2018; Timmermans & Garrett, 2006; Timmermans et al., 2003, 2005; Woodgate et al., 2007). Below the core of the warm Atlantic Water Layer, potential temperature decreases with depth to a minimum at about 2,400 m. Deeper than this temperature minimum, tempera- ©2018. American Geophysical Union. ture increases through a transition layer toward the top of a well-mixed bottom water layer extending from All Rights Reserved. ∼2,750-m depth to the ocean bottom, ∼3,500 m (Figure 3a). The transition layer temperature profile has ZHAO AND TIMMERMANS 1 Journal of Geophysical Research: Oceans 10.1029/2018JC014233 Figure 1. (a) Depth-time series (6 years) of potential temperature ; the mean over 5 days is shown. Measurements are from Beaufort Gyre Observing System Mooring D (located at the red star shown on the inset map) at 74∘N, 140∘W, in the vicinity of a weak bottom slope. Note that the upper 2,000 m and that below are from the shallow and deep McLane Moored Profilers, respectively, and are shown with different color scales. (b) Standard deviation of isopycnal displacements (blue circles) and isotherm displacements (red stars) at selected depths (see text). The black curve is the exponential fit to the profile of isopycnal displacements, with a vertical decay length of ∼1,000 m. Data after 23 August 2012 are excluded from this analysis (see text). a staircase structure (possibly a double-diffusive staircase), a region which has been shown to exhibit both inertial and subinertial motions (Timmermans et al., 2007). Analyzing a yearlong mooring record of deep potential temperature measurements in the Beaufort Gyre, Timmermans et al. (2010) characterized the dominant subinertial frequency to be around 50 days; they found that coherent vertical water column excursions (inferred from the temperature signal) exist through the water column with displacement amplitudes decaying approximately exponentially toward the surface. This is Figure 2. (a) Depth-time series (6 years) of east-west u speed; the mean over 5 days is shown. The north-south speed (not shown) shows similar characteristics. Shallow and deep McLane Moored Profiler data are shown with the same color scale (cf. Figure 1a). (b) The mean profile of potential density anomaly referenced to the surface (black) and the 2 g mean buoyancy frequency (N =− , blue). Potential density is computed by averaging depths of a given isopycnal 0 z for all density profiles before 23 August 2012. The horizontal red line denotes the depth (∼300 m) where N becomes weak and approximately uniform with depth. (c) The wavelet transform of vertically averaged u below ∼300 m. The white shaded cone of influence indicates the maximum period of useful information at that particular time. Periods longer than this are subject to edge effects. The black contour indicates the 5% significance level against red noise. Note the concentration of power between ∼30 and ∼100 days. ZHAO AND TIMMERMANS 2 Journal of Geophysical Research: Oceans 10.1029/2018JC014233 Figure 3. (a) Time-averaged potential temperature as a function of depth. Note that the temperature scale is different for the upper (top x axis) and lower (bottom x axis) portions. (b) Coherency and (c) phase between vertically averaged u-v and over all depths. The black contour in (b) denotes the 95% confidence level for coherency, ∼0.44. To ensure consistency between the shallow and deep calculations, coherency/phase results are presented from a yearlong record for both the shallow and deep Mooring (2011–2012). Extending this for the entire shallow mooring time series yields similar results. typical of TRWs (e.g., Rhines, 1970; Shu et al., 2016; Thompson & Luyten, 1976), which arise from conservation of potential vorticity applying to topographically induced water column stretching/squashing. Cross-slope flows give rise to water column stretching (squashing), which induces anomalous positive (negative) relative vorticity to ensure conservation of potential vorticity. The anomalous relative vorticity field induces further cross-slope motions nearby: The perturbations thus propagate, with shallow water on the right in the North- ern Hemisphere, as TRWs in much the same way as planetary Rossby waves propagate westward under the effect. Adopting reasonable estimates of the ocean bottom slope and stratification structure, Timmermans et al. (2010) derived a TRW dispersion relation for the Canada Basin and showed consistency with the ∼50-day subinertial period characterizing the temperature observations. Here we analyze a longer time series of moored measurements, including horizontal velocity information, to further understand these motions. One dominant feature that our analysis aims to explain is the observation that subinertial horizontal motion is approximately uniform in depth below the strongly stratified halocline (i.e., in the water column deeper than ∼300 m). Approximately barotropic horizontal flow would appear to be inconsistent with the bottom-intensified
Details
-
File Typepdf
-
Upload Time-
-
Content LanguagesEnglish
-
Upload UserAnonymous/Not logged-in
-
File Pages10 Page
-
File Size-