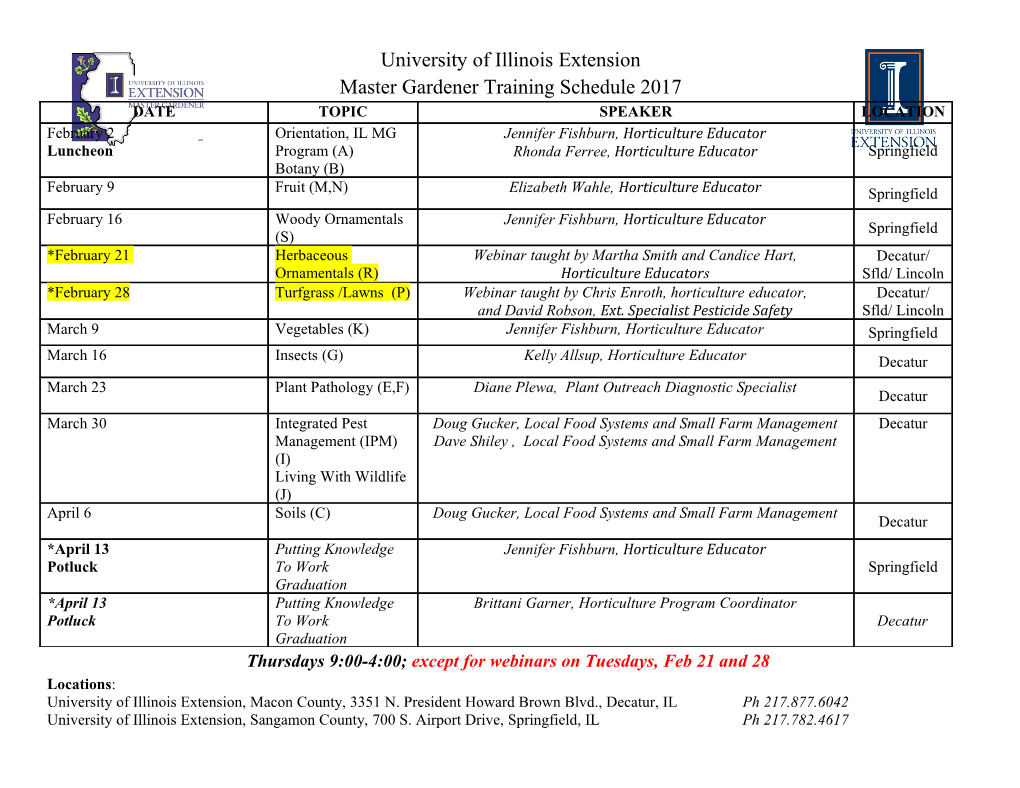
agronomy Article Bacterial Community in Soils Following Century-Long Application of Organic or Inorganic Fertilizers under Continuous Winter Wheat Cultivation Xiufen Li 1,2 , Shiping Deng 1,*, William R. Raun 1, Yan Wang 1 and Ying Teng 3 1 Department of Plant and Soil Sciences, Oklahoma State University, Stillwater, OK 74078, USA; [email protected] (X.L.); [email protected] (W.R.R.); [email protected] (Y.W.) 2 Texas A&M AgriLife Research Center at Beaumont, Texas A&M University System, Beaumont, TX 77713, USA 3 Institute of Soil Science, Chinese Academy of Sciences, Nanjing 210008, China; [email protected] * Correspondence: [email protected]; Tel.: +1-405-744-9591 Received: 30 July 2020; Accepted: 29 September 2020; Published: 1 October 2020 Abstract: Fertilization is one of the most common agricultural practices to achieve high yield. Although microbes play a critical role in nutrient cycling and organic matter decomposition, knowledge of the long-term responses of the soil bacterial community to organic and inorganic fertilizers is still limited. This study was conducted to evaluate the effects of century-long organic (manure), inorganic (NPK), and no fertilization (control) treatments on soil bacterial community structure under continuous winter wheat (Triticum aestivum L.) cultivation. Fertilization treatments altered the richness, diversity and composition of the soil bacterial community. Compared with the control, manure significantly increased the operational taxonomic units (OTUs), Chao 1 and Shannon indices, and taxonomic groups, while NPK significantly decreased these parameters. Fertilization treatments did not alter the types of dominant phyla but did significantly affect their relative abundances. Acidobacteria and Proteobacteria were the most dominant phyla in all treatments. Manure led to enrichment of most phyla, with a diazotrophic group, Cyanobacteria, being an exception; NPK reduced most phyla, but enriched Chloroflexi; control led to promotion of Cyanobacteria. Soil pH and NO3− were two dominant parameters influencing the bacterial community structure. Soil pH positively correlated with the relative abundances of Proteobacteria and Gemmatimonadetes but negatively correlated with those of Acidobacteria and Chloroflexi; NO3− negatively correlated with the relative abundance of Cyanobacteria, which was 14–52 times higher in control than the fertilized soils. Cyanobacteria, especially M. paludosus and L. appalachiana, could be the key players in maintaining wheat productivity in the century-long unfertilized control. Keywords: soil bacterial community; 16S rRNA; organic fertilizer; inorganic fertilizer; manure; NPK 1. Introduction Understanding the soil microbial community structure presents understated challenges, largely due to the complex nature of the system and the enormous abundance and diversity of the community residing within [1]. Bacteria comprise over 70% of the total soil microorganisms [1–3]. With as many as one billion bacterial cells and an estimated 8.3 million species in each gram of soil [4], the challenges in revealing and understanding the richness, diversity and composition of soil microbial community are thought-provoking. Nevertheless, the importance of the soil microbial community in nutrient acquisition, cycling, and availability is amply evidenced [5–8]. Agronomy 2020, 10, 1497; doi:10.3390/agronomy10101497 www.mdpi.com/journal/agronomy Agronomy 2020, 10, 1497 2 of 15 Numerous studies have demonstrated that healthy soil harbors abundant and diverse microbes, while environmental perturbation and management practices could lead to changes in microbial community structure that impact soil health and productivity [5–9]. Although long-term manure application resulted in enrichment of soil organic matter and microbial abundance [5,10–13], it did not always translate into higher crop yield than soils supplemented with inorganic fertilizers [5,14]. Long-term inorganic fertilizer addition, on the other hand, may lead to soil acidification and decreases in microbial abundance and diversity [5,11,13,15]. Based on an analysis of 22 soils from a liming experiment spanning > 60 years, Rousk et al. [10] found that both relative abundance and diversity of bacteria were positively correlated with soil pH in the range from 4.0 to 8.3. In contrast, Lauber et al. [16] found that bacterial richness peaked in the near-neutral pH range based on analysis of 132,088 16S rRNA sequences from 88 soils. The observed inconsistency could, in part, be due to gradual system-level changes that make their detection challenging [7,8,17]. Although the dominant bacterial phyla in soil are reported to be Proteobacteria, Acidobacteria, Actinobacteria, Verrucomicrobia, and Bacteroidetes [18], their relative abundances varied considerably in pristine forest, grassland, winter wheat fields, and rice paddies [13,19–22]. Of soil properties, carbon and N availabilities and pH are recognized as key factors governing changes in the soil bacterial community composition. However, the relative importance of these factors in driving changes in specific bacterial groups that impact ecosystem function and soil productivity is still not clear. A century-long continuous winter wheat (Triticumaestivum L.) experiment provided an opportunity for extending our understanding of the effect of long-term organic and inorganic fertilizations on the bacterial community structure. We hypothesized that different fertilization practices would differentially affect soil properties and bacterial communities over century-long wheat cultivation. Insights gained from long-term studies are crucial to support sustainable wheat production. The specific objectives were to (1) evaluate richness, diversity, and composition of the soil bacterial community in response to century-long organic, inorganic, and no fertilization regimes under continuous winter wheat cultivation; and (2) identify drivers in these soils that govern the richness, diversity and composition of the bacterial community. 2. Materials and Methods 2.1. Site Description and Soil Sampling This study was conducted in a century-long continuous winter wheat (Triticum aestivum L.) experimental field established in 1892 in central Oklahoma, USA (36◦7011” N, 97◦5019” W). The soil is a Kirkland silt loam (fine, mixed, thermic Udertic Paleustolls) with 37.5% sand, 40% silt, and 22.5% clay. Annual precipitation in this region (CD5 Central Oklahoma, OK, USA) ranged from 483 to 1448 mm (average 874 mm) and annual air temperature ranged from 14.4 to 17.7 ◦C (average 15.8 ◦C) based on data from 1895 to 2019 (https://climate.ok.gov). The field was conventionally tilled before planting every year. Comprehensive descriptions of this experimental site, soil properties, and winter wheat yield from 1892–2014 have been reported by Omara et al. [14], Girma et al. [23], and Aula et al. [24]. In this study, soil treatments were: no fertilizer added (Control), organic fertilizer applied in the form of cattle manure (Manure), and inorganic nitrogen, phosphorus, and potassium fertilizers (NPK). The manure treatment was initiated in 1899 and NPK treatment was added in 1929. Cattle manure 1 from a feedlot was applied every four years at 269 kg N ha− and inorganic NPK fertilizers were 1 1 1 applied annually in October before planting at 67 kg N ha− , 14.6 kg P ha− , and 28 kg K ha− in the forms of NH4NO3, Ca(H2PO4)2, and KCl, respectively. When the field experiment was initiated in 1892, the application of statistics to research was not yet firmly established. To compensate for the limited degrees of freedom from replication restrictions, we used an adapted sampling design to obtain three replicated samples from each fertilization treatment and repeated the study in two consecutive years (2012 and 2013). In each year, each treatment plot (30.5 6.1 m) was divided into three subplots (10.2 6.1 m) after wheat harvest. Five soil cores × × Agronomy 2020, 10, 1497 3 of 15 (0–15 cm) were collected from each subplot and composited as one soil sample. A total of 18 composite soil samples were collected for the three treatments in two years. Following sample collection, soils were placed in a cooler with dry ice and transported to the laboratory. Each field-moist sample was then passed through a 2 mm sieve, mixed thoroughly, and divided into two portions. One portion was air-dried and stored at room temperature for chemical analyses, and the other was freeze-dried and stored at 80 C for subsequent biological and genetic analyses. − ◦ Soil pH was determined using a soil: water ratio of 1:2.5 (v/v) and a combination glass electrode (Mettler-Toledo International Inc., Columbus, OH, USA). Soil organic carbon (SOC) and total nitrogen + (TN) were determined using a Carlo-Erba NA 1500 nitrogen/carbon/sulfur analyzer [25]. Soil NH4 and NO3− were extracted using 2 M KCl and analyzed with a BioTek Epoch 2 microplate reader (BioTek Instruments, Inc., Winooski, VT, USA) at OD660 and OD550, respectively. Microbial biomass carbon (MBC) was determined using the chloroform-fumigation incubation method with a Kc factor of 0.45 [26,27]. 2.2. Soil DNA Extraction, PCR Amplification and Sequencing Soil DNA was extracted from 0.5 g soil using an Ultra Clean Soil DNA Isolation Kit (MO BIO Laboratories, Inc., Carlsbad, CA, USA) according to the manufacturer’s instructions. The quality and quantity of DNA was evaluated utilizing agarose gel electrophoresis and a NanoDrop-1000 spectrophotometer (NanoDrop Technologies, Inc., Wilmington, DE, USA). All extracted DNA had an A260/A280 > 1.8 and was of sufficient purity to be used in a polymerase chain reaction (PCR) following a 10-fold dilution with nuclease-free water. PCR primers were 27F (50-AGA GTT TGA TCC TGG CTC AG-30) and 1492R (50-GGT TAC CTT GTT ACG ACT T-30)[28,29]. Each 25 µL PCR reaction mixture contained 1 µL of template DNA at 1 0.25 ng µL− , 1 µL each of the forward and reverse primers at 10 µM, 12.5 µL of Taq 2X Master Mix (New England Biolabs Inc., Ipswich, MA, USA), and 9.5 µL of H2O. After an initial denaturation for 2 min at 94 ◦C, amplification was carried out in 35 cycles with each cycle consisting of denaturation (40 s, 94 ◦C), annealing (40 s, 49 ◦C), and extension (40 s, 72 ◦C).
Details
-
File Typepdf
-
Upload Time-
-
Content LanguagesEnglish
-
Upload UserAnonymous/Not logged-in
-
File Pages15 Page
-
File Size-