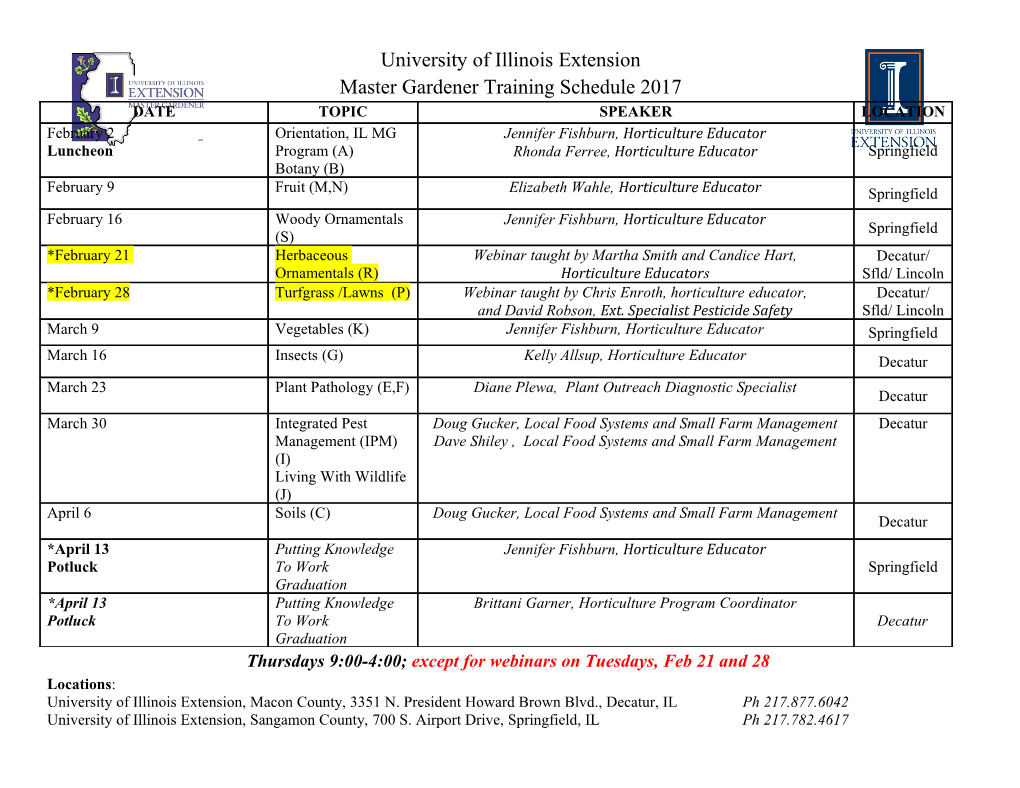
AN ABSTRACT OF THE THESIS OF Beth E. Basham for the degree of Doctor of Philosophy in Biochemistry & Biophysics presented on March 11, 1998. Title: The Analysis and Prediction of DNA Structure. Redacted for Privacy Abstract approved. P. Shing Ho As genome sequencing projects begin to come to completion, the challenge becomes one of determining how to understand the information contained within the DNA. DNA is a polymorphic macromolecule; the A- B- and Z-DNA conformations have been observed by a variety of physical techniques. The magnitude of the energetic differences between these conformations suggests that these conformations may be important biologically and thus relevant in the analysis of genomes. A computer program, NASTE, was developed to evaluate the helical parameters of the set of Z-DNA crystal structures in order to determine the true conformation of Z-DNA and to understand the effects of various factors on the observed structure and stability. A thermodynamic method, elucidated in part with a genetic algorithm, was developed to predict the sequence-dependent propensity of DNA sequences for A- versus B-DNA in both the crystal and in natural DNA. Predictions from this method were tested by studying the conformation of short oligonucleotides using circular dichroism spectroscopy. Finally, the thermodynamic method was applied in an algorithm, AHUNT, to identify regions in genomic DNA with a high propensity to form A-DNA. Significant amounts of A-DNA were identified in eukaryotic and archeabacterial genes. E. coli genes have less A-DNA than would be predicted from their (G+C) content. These results are discussed with respect to the intracellular environment of the genomes. © Copyright by Beth E. Basham March 11, 1998 All Rights Reserved THE ANALYSIS AND PREDICTION OF DNA STRUCTURE by Beth E. Basham A THESIS submitted to Oregon State University in partial fulfillment of the requirements for the degree of Doctor of Philosophy Completed March, 11 1998 Commencement June 1998 Doctor of Philosophy thesis of Beth E. Basham presented on March 11, 1998 APPROVED: Redacted for Privacy Major .ofessor, representing Biochemistry & Biophysics Redacted for Privacy Chair of Department of Biochemistry & Biophysics Redacted for Privacy Dean of Gradua Schoolchooll I understand that my thesis will become part of the permanent collection of Oregon State University libraries. My signature below authorizes release of my thesis to any reader upon request. Redacted for Privacy Beth E. Basham, Author ACKNOWLEDGMENT The completion of this work would not have been possible without the help and support of many special people. I value the guidance, support and acute scientific insight of Dr. P. Shing Ho, who provided numerous wonderful opportunities and taught me how to ask the right questions and seek out the answers. I must also acknowledge the past and present members of the Ho lab: Dr. Gary P. Schroth for the all advice and the critical evaluation of this work and my ideas, and fellow students Dr. Todd Kagawa, Dr. Blaine Mooers, Brandt Eichman and Jeff Vargason for their help and friendship. The circular dichroism would not have been possible without the expert technical advice of Dr. W. Curtis Johnson and Jeannine Lawrence. The contribution of the faculty at Oregon State University was also vital to this work, particularly the people involved in its evolution and evaluation: Dr. Michael Schimerlik, Dr. Philip McFadden, Dr. Victor Hsu and Dr. Mark Christensen. I would especially like to thank Dr. Victor Hsu for his helpful and patient discussions about DNA solution structure and Dr. Kensal van Holde for his interest in this work. I also value the personal and professional friendships of Monika Ivancic, Debbie Mustacich, Indira Rajagopal, Kevin Ahern, Cyndi Thompson, and Laura Meek. None of this would have been possible without the continuous support of my parents, Pat and John Etchells, my brother, Sean, and my grandparents, Ray and Edna Etzold. Finally, I am very thankful to Eric, my husband and my muse, for his unfaltering patience and inspiration. CONTRIBUTION OF AUTHORS Brandt Eichman assisted in the analysis of the Z-DNA crystal structures, in the compilation of the tables and in the preparation of the review (Chapter 2). He also analyzed the set of Z-DNA sequences for length effects. Dr. P. Shing Ho described the solvent structure of d(CGCGCG). Dr. Gary P. Schroth assisted in the design of the circular dichroism experiments and designed and collected the data for some of the oligonudeotide sequences presented here (Chapter 3, 4). Dr. P. Shing Ho guided all aspects of the projects described here. TABLE OF CONTENTS 1. INTRODUCTION 1 1.1 Biological functions of DNA that are defined by its structure 3 1.2 DNA structure 4 2. THE SINGLE-CRYSTAL STRUCTURES OF Z-DNA 14 2.1 Synopsis 15 2.2 Introduction 15 2.3 The prototypical Z-DNA structure of d(CGCGCG) 17 2.3.1 The structure of Z-DNA 20 2.3.2 The helix structure of d(CGCGCG) 31 2.3.3 The solvent structure of d(CGCGCG) 37 2.3.4 Cation effects on the structure of d(CGCGCG) 43 2.3.5 Length effects on the structure of d(CpG) sequences as Z-DNA 56 2.4 Sequence and substituent effects on the structure and stability of Z-DNA 66 2.4.1 Effects of cytosine methylation on Z-DNA structure 69 2.4.2 Effects of cytosine bromination on Z-DNA structure 81 2.4.3 Effects of the N2-amino of guanine on the structure and stability of Z-DNA 83 2.4.4 The structure and stability of d(TpA) dinucleotides in Z-DNA 85 2.4.5 d(CpA)/d(TpG) dinucleotides in Z-DNA 97 2.4.6 Out-of-alternation structures 100 2.5 Summary--Sequence effects on the structure and stability of Z-DNA 110 2.6 Acknowledgments 120 3. AN A-DNA TRIPLET-CODE: THERMODYNAMIC RULES FOR PREDICTING A- AND B-DNA 121 3.1 Synopsis 122 3.2 Introduction 122 TABLE OF CONTENTS (continued) 3.3 Materials and Methods 125 3.3.1 SFE calculations: 125 3.3.2 Solution studies: 127 3.4 Results 127 3.4.1 A- and B-DNA data sets 127 3.4.2 Distinguishing A- and B-DNA by SFEs 129 3.4.3 A triplet code to predict A-DNA formation 134 3.4.4 APE predictions for A- and B-DNA in crystals 136 3.4.5 Conformations of oligonucleotides in solution 137 3.5 Discussion 143 3.6 Acknowledgments 145 4. THE IDENTIFICATION OF A-DNA IN GENOMIC DNA SEQUENCES 146 4.1 Synopsis 147 4.2 Introduction 148 4.3 Methods 156 4.3.1 Calculation of ASFEA_B 156 4.3.2 Genetic algorithm 157 4.3.2.1 Population 158 4.3.2.2 Fitness 162 4.3.2.3 Recombination 164 4.3.2.4 Mutation 164 4.3.2.5 Culling the population 164 4.3.2.6 Termination 165 4.3.3 TFE titration of DNA oligonucleotides 165 4.3.4 AHUNT 166 4.3.5 The application of AHUNT to analysis of genomic DNA 170 4.4 Results 170 4.4.1 The genetic algorithm identifies a set of APEs that reflect both the SFE and the UV footprinting information 174 4.4.1.1 Description of APE trends 178 4.4.1.2 The APEs correlate with the titration behavior of short oligonucleotides in solution 182 TABLE OF CONTENTS (continued) 4.4.2 AHUNT: an application of the APEs to predict gene structure 184 4.4.2.1 Testing and calibration of AHUNT with the 5S gene 184 4.4.2.2 A-DNA is not localized to a specific part of human genes 186 4.4.2.3 Analysis of genes from different species 190 4.5 Discussion 197 5. SUMMARY 204 5.1 The systematic evaluation of Z-DNA structure 205 5.2 Development of a general predictive method for A-DNA sequence- dependent stability 210 5.3 Testing the A-DNA propensity energies 214 5.4 The application of the A-DNA propensity energies to identifying A-DNA in genomes 217 BIBLIOGRAPHY 225 LIST OF FIGURES Figure rage 1.1 The A-, B- and Z-conformations of DNA 9 2.1 Structure of d(CGCGCG) as Z-DNA 25 2.2 Comparison of the guanine nucleotide in the syn conformation (A) and cytosine in the anti conformation (B) of d(CGCGCG) as Z-DNA 27 2.3 Helical parameters calculated with NASTE 33 2.4 Solvent interactions with d(CGCGCG) as Z-DNA 40 2.5 Comparison of the cation interactions between the magnesium only (MG), mixed magnesium/spermine (MGSP), spermine only (SP), and mixed magnesium/spermidine (MGSD) forms of d(CGCGCG) 48 2.6 Definitions and structures of variations in d(C0G) and d(TA) type base pairs 67 2.7 Titration of unmethylated, methylated and hemimethylated d(CpG) dinucleotides with MgC12 to induce the fomation of Z-DNA 78 2.8 Comparison of the solvent structures and widths of the minor groove crevice of d(UpA) dinucleotides in Z-DNA 94 2.9 Comparison of the out-of-alternation bases in the structures of d(m5CGATm5CG) and d(m5CGGGm5CG)/d(m5CGm5CCm5CG) 105 2.10 Effect of substituent groups on the differences in solvent free energies (ASFE) and the stability (AAG°T) of dinucleotides in Z-DNA versus B-DNA 116 LIST OF FIGURES (continued) Figure Page 2.11 The relationship between the effective cation concentration of the crystallization solutions and the difference in solvent free energy between Z-DNA and B-DNA (AASFEz_B) for sequences crystallized as Z-DNA 116 3.1 Distributions of ASFEA_B for A-DNA (top) and B-DNA (bottom) sequences 130 3.2 The A-DNA triplet code of A-DNA propensity energies (APEs) 135 3.3 CD spectra of DNA dodecanucleotides titrated with TFE 141 4.1 The percent 1PE at the midpoint of the B-to-A transition (TFErnid) for residues in the 5S rRNA gene as measured by UV photofootprinting 155 4.2 Schematic of the genetic algorithm 159 4.3 Recombination produces new solutions 160 4.4 The determination of A- and B-DNA regions using AHUNT 168 4.5 Summary of genetic algorithm runs 175 4.6 The APEs calculated with the genetic algorithm predict TFEmid for 11 regions of the 5S rRNA gene 179 4.7 The A-DNA triplet code of the A-DNA propensity energies (APEs) 181 4.8 AHUNT's predictions correlate with the observed TFEmid for the 5S rRNA gene 185 4.9 Distributions of characteristics of A-DNA regions in four sections of genes for the set of 154 human genes 187 LIST OF FIGURES (continued) Figure Page 4.10 A-DNA, B-DNA and a/b-DNA content of 154 human genes versus the (G+C) content of the gene 192 4.11 A-DNA, B-DNA and a/b-DNA content of 104 E.
Details
-
File Typepdf
-
Upload Time-
-
Content LanguagesEnglish
-
Upload UserAnonymous/Not logged-in
-
File Pages257 Page
-
File Size-