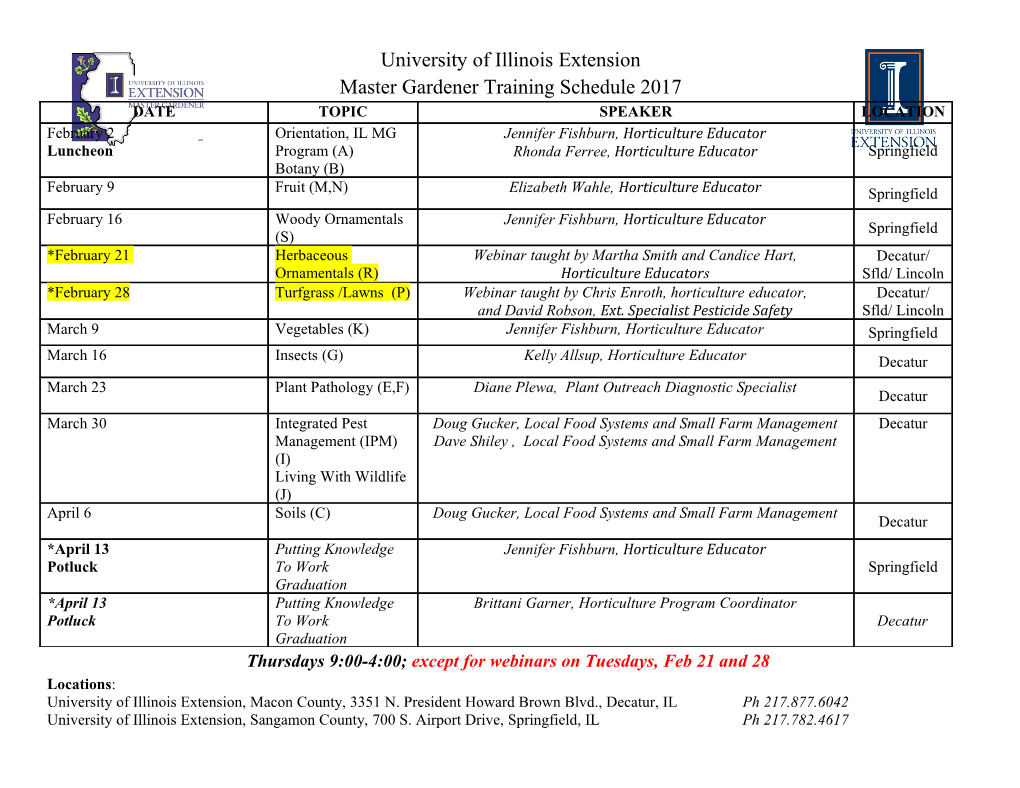
C O L L O Q U I U M M A T H E M A T I C U M VOL. 92 2002 NO. 1 ON SOME PROBLEMS OF MĄKOWSKI{SCHINZEL AND ERDOS} CONCERNING THE ARITHMETICAL FUNCTIONS φ AND σ BY FLORIAN LUCA (Morelia) and CARL POMERANCE (Murray Hill, NJ) Abstract. Let σ(n) denote the sum of positive divisors of the integer n, and let φ denote Euler's function, that is, φ(n) is the number of integers in the interval [1; n] that are relatively prime to n. It has been conjectured by M¡kowski and Schinzel that σ(φ(n))=n ≥ 1=2 for all n. We show that σ(φ(n))=n ! 1 on a set of numbers n of asymptotic density 1. In addition, we study the average order of σ(φ(n))=n as well as its range. We use similar methods to prove a conjecture of Erd}os that φ(n − φ(n)) < φ(n) on a set of asymptotic density 1. 1. Introduction. In this paper, we investigate a couple of conjectures concerning inequalities involving the arithmetical functions φ, σ, and com- positions of these. As usual, for a positive integer n we write φ(n) for the Euler function of n, and σ(n) for the sum-of-divisors function of n. For any positive integer k and any positive number x we define logk(x) recursively as the maximum of 1 and log(logk−1(x)), where log1 = log is the natural loga- rithm. Throughout this paper, we use p, q and P to denote prime numbers, and c1; c2; : : : will denote positive computable constants. The first conjecture we are looking at is due to M¡kowski and Schinzel (see [18]) and asserts that the inequality σ(φ(n)) 1 (1) n ≥ 2 holds for all positive integers n. It is known that σ(φ(n)) σ(φ(n)) 1 1 (2) lim sup = and 0 < lim inf + 34 : n n 1 n n ≤ 2 2 1 − The first limit in (2) is due to Alaoglu and Erd}os (see [1]). The positive lower bound for the second limit in (2) is due to the second author (see [20]) and the upper bound for the same limit appears in the original paper of M¡kowski and Schinzel [18]. It is known that (1) holds for positive integers n of various shapes (see, for example, [2], [6], [11], [18]), and in fact in [1] it 2000 Mathematics Subject Classification: 11A25, 11N37, 11N56. Key words and phrases: sum-of-divisors function, Euler's function. [111] 112 F. LUCA AND C. POMERANCE is asserted that σ(φ(n))=n on a set of asymptotic density 1, and that σ(φ(n))/φ(n) eγ log (n) !on 1a set of asymptotic density 1. ∼ 3 Especially since the proofs of these results stated in [1] were not given, and since later researchers have labored to give somewhat weaker results (in [15] it is shown that (1) holds on a set of lower density at least 0:74), we think it is worthwhile to prove these assertions from [1], in a somewhat stronger form. For every positive integer n we write S(n) := σ(φ(n))=n. Our first result in this paper gives the maximal, normal, and average orders of the function S(n). Theorem 1. (i) We have S(n) (3) lim sup = eγ : n log2(n) (ii) For each number u, 0 u 1, the asymptotic density of the set of numbers n with ≤ ≤ γ (4) S(n) > ue log3(n) exists, and this density function is strictly decreasing, varies continuously with u, and is 0 when u = 1. (iii) For every positive number x, 1 6eγ (5) S(n) = log (x) + O((log (x))1=2): x π2 · 3 3 1≤Xn≤x We remark that Warlimont (see [22]) has recently shown a result similar to part (iii) of Theorem 1 for the function φ(n)/φ(φ(n)). There are several results in the literature which assert that if f(n) is, for example, either of the functions σ(n)=n or φ(n)=n, then the closure of the set f(n) n≥1 is an interval. Our next result shows that the same is true for the ffunctiong f(n) = S(n). Theorem 2. Let α = lim inf n S(n). Then S(n) n 1 is dense in the interval [α, ]. f j ≥ g 1 We now leave the function S(n) and the next question we address is a conjecture of Erd}os (see [8]) which asserts that the inequality (6) φ(n φ(n)) < φ(n) − holds on a set of positive integers n of asymptotic density 1 (i.e., for almost all positive integers n), but the inequality (7) φ(n φ(n)) > φ(n) − holds for infinitely many positive integers n. Some infinite families of positive integers n for which inequality (7) holds were pointed out in [16]. In that paper, it was also shown that (6) holds for a set of positive integers n of lower PROBLEMS OF MĄKOWSKI{SCHINZEL AND ERDOS} 113 density at least 0.54. In this note, we prove that (6) holds indeed for almost all positive integers n. In fact, we prove a stronger statement, namely: Theorem 3. (i) Let "(x) be any positive function of the positive vari- able x which tends to zero when x tends to infinity. The set of integers n > 1 for which the inequality (8) φ(n φ(n)) < φ(n) n "(n) − − · fails has asymptotic density 0. (ii) The set of positive integers n for which the inequality φ(n) φ(n φ(n)) 2 log (n) (9) − < 3 n − n φ(n) log (n) 2 − fails has asymptotic density 0. For example, (9) implies that for any fixed " > 0 the set of n for which φ(n) φ(n φ(n)) − < " n − n φ(n) − fails has asymptotic densit y 0. In particular, the two functions φ(n)=n and φ(n φ(n))=(n φ(n)) are asymptotically equal on a set of n of asymptotic densit−y 1. − For n > 1, let f(n) = φ(n φ(n))/φ(n). It can be shown, using the method of proof of Theorem 2,−that the set of numbers f(n) is dense in the interval [0; ]. This result shows, in particular, that if c is any positive number, then the1 inequality (10) φ(n φ(n)) > cφ(n) − holds for infinitely many positive integers n, which is a statement much stronger than the fact that (7) holds for infinitely many positive integers n. We do not give further details here. 2. Preliminary results. In 1928, Schoenberg (see [21]) proved that the function φ(n)=n has a distribution. That is, D(u) defined as the asymptotic density of the set of n with φ(n)=n u exists for every u. In addition, D(u) is continuous and strictly decreasing≥ on [0; 1]. Clearly, D(0) = 1 and D(1) = 0. From these considerations, we immediately derive the following: Lemma 1. Let " : (0; ) (0; 1) be any function such that "(x) tends to zero as x tends to infinity.1 !Then, for a set of n of asymptotic density 1, φ(n) (11) > "(n): n Proof. This is almost obvious. Indeed, let " be an arbitrarily small posi- tive number. For large x we have "(x) < ". Thus, the set of positive integers n for which inequality (11) holds contains a set of n of asymptotic density 114 F. LUCA AND C. POMERANCE at least D("). Since this holds for every " > 0, it follows that inequality (11) holds for a set of n of asymptotic density D(0) = 1. Lemma 1 may also be proved by using the average order of φ(n)=n, namely, φ(n) 6 (12) = x + O(log(x)); n π2 nX≤x but we shall have other uses for the distribution function D(u) later. The next result plays a key role in the proofs of both Theorems 1 and 3 and is essentially due to Erd}os, Granville, Pomerance and Spiro (see [9]). However, since this result was not explicitly stated in [9], we state it below and provide a short proof. Lemma 2. There exists a computable positive constant c1 such that on a set of n of asymptotic density 1, φ(n) is divisible by all prime powers pa a with p < c1 log2(n)=log3(n). Proof. We use the notations from [9]. For any positive integer m and any positive number x we let 1 (13) S(x; m) = : q qX≤x mj(q−1) From Theorem 3.4 in [9], we know that there exist computable positive numbers c1; x0 such that the inequality c log (x) (14) S(x; m) > 1 2 φ(m) holds for all x > x0 and all m log(x). Let g(x) = c1 log2(x)=log3(x). From the proof of Theorem 4.1 in [9],≤ we have, uniformly for all m, c x (15) 1 < 2 : exp(S(x; m)) nX≤x m-φ(n) a a Assume now that p is any prime power with p < g(x). By increasing x0 if necessary, we assume that the inequality log(x) > g(x) holds for x > x0. By inequalities (14) and (15), it follows that for such pa, c x c x c x (16) 1 < 2 < 2 = 2 : exp(S(x; pa)) exp (log (x)) log (x) nX≤x 3 2 pa-φ(n) Now let M(x) be the least common multiple of all the prime powers pa<g(x).
Details
-
File Typepdf
-
Upload Time-
-
Content LanguagesEnglish
-
Upload UserAnonymous/Not logged-in
-
File Pages20 Page
-
File Size-