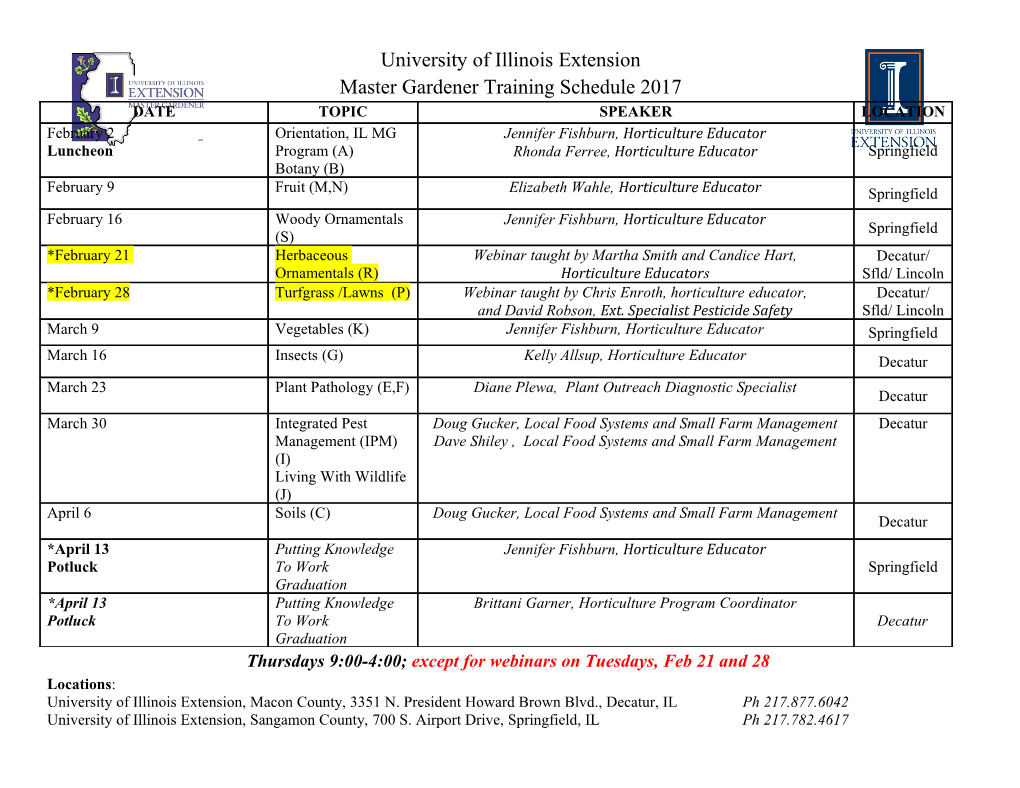
ABSTRACT MOLECULAR MODELING OF IONIC LIQUIDS FOR POTENTIAL APPLICATIONS IN THE DESULFURIZATION OF DIESEL FUEL by Miranda R. Caudle The sulfur compounds in diesel fuel produce harmful environmental pollutants during combustion. Hydrodesulfurization (HDS) is the most common technique to reduce the sulfur content of diesel fuel but cannot effectively remove the aromatic sulfur compounds to produce ultra-low-sulfur diesel. Ionic liquids (ILs) show potential as alternative solvents for extractive desulfurization to be implemented after a conventional HDS process, but the mechanism is not well understood. This work focuses on using a combination of free energy calculations and detailed structural analysis to better understand the molecular-level interactions between dibenzothiophene and seven common imidazolium-based ILs. The free energy calculations suggest that the ILs interact differently with thiophene and dibenzothiophene. No specific interactions were observed between the anion and dibenzothiophene; varying the anion showed no remarkable differences in the observed interactions. It was determined that interactions between dibenzothiophene and the cation were more significant; π-π stacking between the imidazole ring and thiophene ring plus electrostatic interactions between the alkyl chain and benzene rings were observed. The primary goal of this work was to use molecular dynamic simulations to complement current experimental research to find a suitable IL for potential desulfurization applications. A Thesis Submitted to the Faculty of Miami University in partial fulfillment of the requirements for the degree of Master of Science by Miranda R. Caudle Miami University Oxford, Ohio 2018 Advisor: Dr. Andrew Paluch Reader: Dr. Catherine Almquist Reader: Dr. Justin Saul ©2018 Miranda R. Caudle This Thesis titled MOLECULAR MODELING OF IONIC LIQUIDS FOR POTENTIAL APPLICATIONS IN THE DESULFURIZATION OF DIESEL FUEL by Miranda R. Caudle has been approved for publication by The College of Engineering and Computing and Department of Chemical Engineering ____________________________________________________ Dr. Andrew Paluch ______________________________________________________ Dr. Catherine Almquist _______________________________________________________ Dr. Justin Saul Table of Contents Background and Introduction ............................................................................................. 1 Methodology ....................................................................................................................... 7 Computational Details ........................................................................................................ 9 Force Fields ................................................................................................................................. 9 Molecular Dynamics ................................................................................................................. 10 Free Energy Calculations .......................................................................................................... 12 Structural Analysis .................................................................................................................... 14 Results and Discussion ..................................................................................................... 17 Free Energy Calculations .......................................................................................................... 17 Structural Analysis .................................................................................................................... 19 Conclusions ....................................................................................................................... 25 References ......................................................................................................................... 26 Appendix I: Additional Simulation Details ...................................................................... 33 Appendix II: Additional Structural Analysis .................................................................... 37 iii List of Tables Table 1. Summary of the computed dimensionless chemical potential of dibenzothiophene and thiophene in the studied ILs with 0.8 charge scaling along with the computed IL/n-decane partition coefficient ...................................................................... 17 iv List of Figures Figure 1. The chemical structure of the solutes – thiophene and dibenzothiophene .......... 8 Figure 2. The chemical structure of the selected anions and imidazolium cation that constitute the seven ionic liquids of interest in this work ................................................... 8 Figure 3. Atom numbering scheme generated by TRAVIS for anion, cation, and solute molecules .......................................................................................................................... 15 Figure 4. RDFs of cation-anion interactions for studied ILs with 0.1 mol frac solute ..... 19 + - Figure 5a. RDFs of solute-anion interactions for [BMIM] [CH3SO4] ............................ 21 + - Figure 5b. RDFs of solute-cation interactions for [BMIM] [CH3SO4] ........................... 21 + - Figure 6. CDFs of solute-cation interactions for [BMIM] [CH3SO4] ............................. 23 - + Figure 7. SDF for dibenzothiophene (red) and [CH3SO4] (blue) around [BMIM] . ....... 24 v MOLECULAR MODELING OF IONIC LIQUIDS FOR POTENTIAL APPLICATIONS IN THE DESULFURIZATION OF DIESEL FUEL Background and Introduction Crude oil products contain sulfur compounds as impurities that are directly related to the emission of sulfur dioxide and sulfur particulate matter when the fuel is burned, which endangers public health and reduces the life of the engine. Research for more efficient and more effective processes to remove these sulfur compounds to reduce sulfur emissions has increased as regulations on the sulfur content of crude oil products have become more stringent [1,2]. Diesel fuel contained as much as 5,000 ppm before the Environmental Protection Agency (EPA) began regulating sulfur content in 1993. In the United States, the allowed sulfur content of diesel fuel has recently been reduced from 500 to 15 ppm and is expected to continue to decrease [3]. Although the percentage of energy obtained from fossil fuels (crude oil, natural gas, and coal) has decreased over recent years, fossil fuels still accounted for 80% of total energy consumption in 2017, and the total energy consumption in the United States continues to increase [4]. Energy obtained from crude oil products has also increased over the last few years and is the source of almost half (46.3%) of fossil fuel consumption in the United States. Crude oil is a complex mixture of organic liquids that is refined to be used mostly as transportation fuels such as gasoline, diesel, and jet fuels. The amount of naturally occurring sulfur compounds present in crude oil depends on the source, but sulfur compounds are generally undesirable in the refining process because they can cause corrosion in the pipelines and refining equipment [1]. Any sulfur compounds left in fuel after processing tend to decrease the effectiveness of catalytic converters over time, resulting in increased emission levels from the vehicle [5]. During the combustion process, these sulfur compounds are oxidized and emitted as sulfur oxide gases (SOx), which react with water in the atmosphere to form sulfates and acid rain. 1 The combustion process also produces sulfate PM that can cause lung cancer and aggravate existing respiratory and cardiovascular diseases. Furthermore, sulfate PM can accumulate on the surface of the catalysts in the exhaust emission control system, reducing the effectiveness of the reactions that remove carbon monoxide (CO), nitrogen oxide gases (NOx), and volatile organic compounds (VOCs). Sulfur-containing fuels generate harmful pollutants during the combustion process that lead to environmental concerns such as smog, global warming, and air and water pollution, even if the sulfur content is below acceptable levels. The naturally occurring sulfur compounds present in diesel fuel are generally categorized into four groups: thiols (R – SH), sulfides (R – S – R’), disulfides (R – S – S – R’), and thiophenes [6,7]. Hydrodesulfurization (HDS) is one of the most common methods used to remove these sulfur compounds and reduce the sulfur content of diesel fuel. Conventional HDS techniques can effectively remove thiols and sulfides but are limited by the aromatic sulfur compounds that remain such as thiophene, benzothiophene, dibenzothiophene, and their alkylated derivatives (especially 4,6-dimethyl dibenzothiophene) [8-10]. During the HDS process, the sulfur compounds are reacted with hydrogen gas (3-5 MPa) at high temperatures (300-450 °C) in the presence of specific catalysts to form hydrogen sulfide gas (H2S), which can be captured and converted into elemental sulfur [1,11]. Operating at higher temperatures and pressures, using more active catalysts, or employing longer residence times can increase the effectiveness of HDS in the removal of aromatic sulfur compounds but are costly to refineries in terms of capital investment and operating costs [2,12,13]. Conventional HDS techniques are not able to produce ultra-low-sulfur diesel (ULSD) while maintaining other fuel requirements [7]. With the ultimate goal of zero-sulfur fuels in mind, there is some research looking at developing new catalysts
Details
-
File Typepdf
-
Upload Time-
-
Content LanguagesEnglish
-
Upload UserAnonymous/Not logged-in
-
File Pages48 Page
-
File Size-