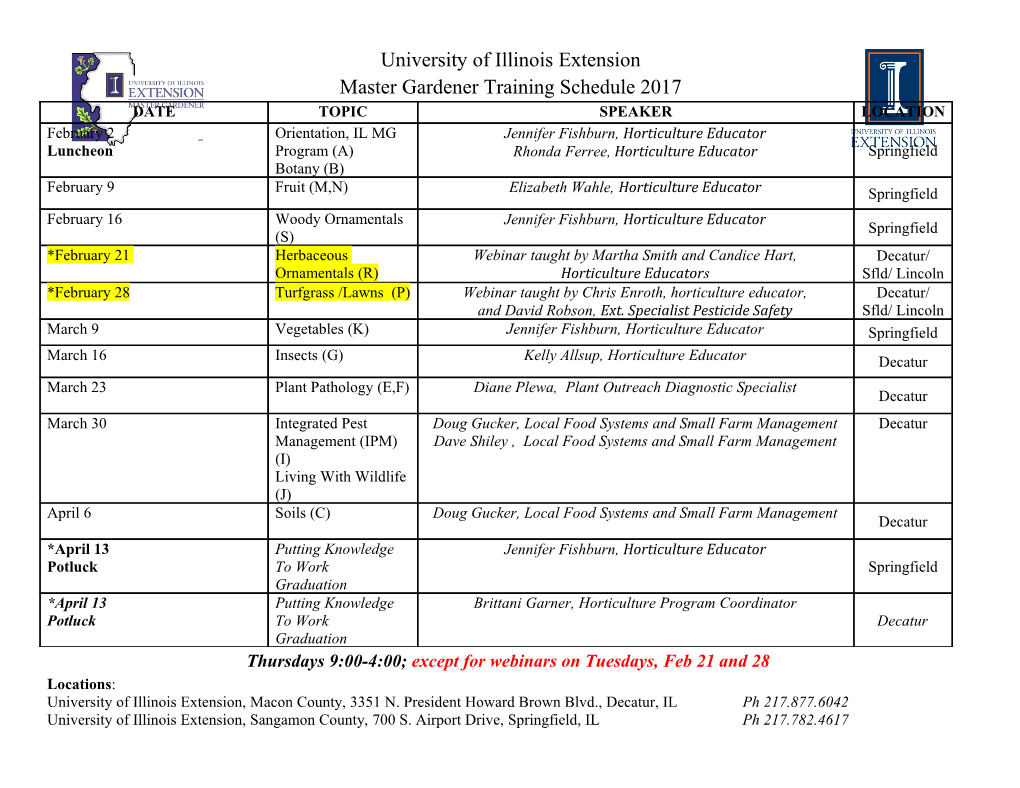
Total Vertical Uncertainty (TVU) Modeling for Topo-Bathymetric LIDAR Systems Firat Eren, Jaehoon Jung, Christopher E. Parrish, Nicholas Sarkozi-Forfinski, and Brian R. Calder Abstract Vertical Uncertainty (TVU) and Total Horizontal Uncertainty This paper presents a comprehensive total vertical uncer- (THU) components and are already widely used for acous- tainty (TVU) model for topo-bathymetric Light Detection and tic survey systems, such as multibeam echosounders. Ranging (LIDAR) systems. The TVU model consists of a combi- Semiautomated data processing algorithms such as Combined nation of analytical uncertainty propagation for the subaerial Uncertainty and Bathymetry Estimator (CUBE) and CUBE with (above water) portion and Monte Carlo simulation models Hierarchical Resolution Techniques are well established and for the subaqueous portion (water surface to seafloor). The accepted as part of standard hydrographic data processing TVU model was tested on a topo-bathymetric LIDAR data set protocols (Calder and Mayer 2003; Calder and Rice 2017), collected by National Oceanic and Atmospheric Administra- being used primarily for acoustic echo-sounding data, but tion’s National Geodetic Survey (NGS) in Southwest Florida, require an estimate of uncertainty be provided with the data U.S., in May 2016 using a Riegl VQ-880-G topo-bathymetric for operation. Bathymetric and topo-bathymetric LIDAR have LIDAR system. The TVU values were compared against the lagged behind acoustic surveying technologies in terms of the empirical standard deviation and were found to capture the development and operational use of TPU models, and there- variability of uncertainty with depth while providing (slightly) fore also with respect to processing improvements. conservative estimates of uncertainty. The results may be Uncertainty propagation in bathymetric LIDAR systems has used to inform data acquisition protocols and data processing been a significant research interest in recent years, and several models. The model implementation is now beginning to be approaches have been proposed. Gonsalves (2010) predicted used operationally at NGS for topo-bathymetric LIDAR projects. uncertainties in LIDAR point clouds for the Coastal Zone Mapping and Imaging LIDAR using the general law of propa- gation of variance (Wolf and Ghilani 1997). However, the Introduction challenge in that approach is that the technical details of the The National Oceanic and Atmospheric Administration’s LIDAR-processing routines from commercial system providers (NOAA) National Geodetic Survey (NGS) Remote Sensing are typically not available (Kinzel et al. 2013), which com- Division (RSD) and Joint Airborne Light Detection and Ranging plicates determining a valid measurement model. Lockhart (LIDAR) Bathymetry Technical Center of Expertise (JALBTCX) et al. (2008) proposed a depth variance method as a proxy partner agencies routinely collect topo-bathymetric LIDAR data for an analytical TPU model. However, the interaction of the Delivered by Ingenta for large portions of the U.S. coast. The data support applica- laser beam with the environment, such as the water surface tions ranging from mapping the national shorelineIP: 192.168.39.210 to regional On:and Fri,the 24water Sep column, 2021 06:58:27 is not accounted for in this approach. Copyright: American Society for Photogrammetry and Remote Sensing sediment management, flood risk management, emergency The effect of the environment on the bathymetric LIDAR response, as well as inland water mapping applications such as measurement uncertainty has been investigated in a few stud- monitoring river morpho-dynamics and instream habitat mod- ies. These studies mainly focused on the effect of the water eling (Wozencraft and Lillycrop 2006; Pfennigbauer et al. 2011; surface on the bathymetric LIDAR measurement accuracy. The Mandlburger et al. 2015; Miller-Corbett 2016; Pan et al. 2016; laser beam refraction angle standard deviation just below the Parrish et al. 2016). The topo-bathymetric LIDAR data could also water surface in along-wind and cross-wind directions has be used to advantage in hydrographic surveying programs, pro- been reported to be in the range of 3–5° (Birkebak et al. 2018). viding valuable information in the shallow nearshore (Imahori Karlsson (2011) also investigated the effect of varying sea- et al. 2013) and supporting the multi-use objective of the state conditions on the bathymetric LIDAR measurement accu- Integrated Ocean and Coastal Mapping initiative (NSTC 2013). racy. The results showed that increasing wind speeds lead to To do so, however, requires reliable, quantitative analysis lower measurement accuracy as demonstrated in simulation and reporting of the spatial coordinate uncertainty of the and empirical measurements. Westfeld et al. (2017) inves- data. According to International Hydrographic Organization tigated the effect of refraction due to ocean waves on LIDAR (IHO) S-44 standards, Total Propagated Uncertainty (TPU) bathymetry coordinates. The results suggested that lateral must account for “all contributing measurement uncertain- coordinate errors can be up to several decimeters as a result ties” using a “statistical method, combining all uncertainty of the refraction pattern. It has been suggested (Steinvall and sources, for determining positioning uncertainty at the 95% Kappari 1996) that maximum horizontal and vertical position confidence level” (IHO 2008). TPU models comprise Total errors are correlated with depth in strong wind speeds that range between 10–12 m/s. Horizontal errors were estimated to Firat Eren and Brian R. Calder are with the Center for Coastal be within 5–10% of depth, and vertical errors were assumed and Ocean Mapping, University of New Hampshire, 24 to be 1–2% of depth. Although these studies investigated Colovos Road, Durham, NH 03824 ([email protected]). the effect of the water surface on bathymetric LIDAR measure- ments, other sources of uncertainty, such as the trajectory Jaehoon Jung, Christopher E. Parrish, and Nicholas Sarkozi- Forfinski are with Oregon State University Civil and Construc- Photogrammetric Engineering & Remote Sensing tion Engineering, 101 Kearney Hall, Corvallis, OR 97331. Vol. 85, No. 8, August 2019, pp. 585–596. Nicholas Sarkozi-Forfinski is also with the National Oceanic 0099-1112/19/585–596 and Atmospheric Administration, National Geodetic Survey, © 2019 American Society for Photogrammetry Remote Sensing Division (Data Solutions and Technology and Remote Sensing Inc.), 1315 East West Highway, Silver Spring, MD 20910. doi: 10.14358/PERS.85.8.585 PHOTOGRAMMETRIC ENGINEERING & REMOTE SENSING August 2019 585 obtained from the Global Navigation Satellite System (GNSS) Methodology aided Inertial Navigation System (INS) and the water column, were not included in the analyses. Carr (2016) proposed an Survey Site and the Data Set The topo-bathymetric LIDAR data used in this study were analytical TPU model to account for the uncertainties due to collected by NOAA NGS with a Riegl VQ-880-G system in the air-water interface and laser path trajectory. Nevertheless, Southwest Florida (vicinity of Cape Romano, Marco Island, the proposed approach relies on the high resolution Digital and Gullivan Bay) in May 2016 (Figure 1). The nominal Surface Model of the water surface generated from the three- operating altitude of the aircraft was 600 m with beam dimensional (3D) infrared (IR) camera device synchronized divergence ranging from 0.7 to 2.0 mrad. The field-of-view with the bathymetric LIDAR, and does not take into consider- for the system is 20°. The bathymetric channel of the LIDAR ation the uncertainty contribution from the water column. ± system (532 nm) employs a rotating prism to provide a cir- This study employs a TVU model that accounts for all the cular scanning pattern with scan speed ranging from 10 to main component uncertainties, including those that arise both 80 rev/s and angular step width ranging from 0.007 to 0.052°. above and below the water surface, as well as at the air-water The data were acquired with a pulse repetition frequency of interface and the water column. The contributions from the 145 kHz, which corresponds to an average point density of subaerial (above water) and subaqueous (water surface to 9 points/ m2. The flight speed during the survey was approxi- seafloor) models are computed separately and then combined mately 220 km/h. The survey site is approximately 193 km2, to produce per-point LIDAR vertical uncertainty values, i.e. TVU with a total of 1.5 billion bathymetric data points ranging from values are generated for the LIDAR points that are identified as -18.7 to -30.5m ellipsoidal height with depths ranging from 0 seafloor points. The prefix “sub” is used here to signify that to 12 m relative to mean lower low water. these portions are two sub-models that compose the TVU model developed in this study. The results provide insight into the General Approach spatially-varying seafloor elevation uncertainty and may be use- The topo-bathymetric LIDAR TVU model is broken into two ful in informing data acquisition and processing procedures. main components, as depicted in Figure 2: the subaerial vec- tor from the LIDAR to the water surface and the subaqueous Delivered by Ingenta IP: 192.168.39.210 On: Fri, 24 Sep 2021 06:58:27 Copyright: American Society for Photogrammetry and Remote Sensing Figure 1. 1 m resolution digital elevation model created from the data points classified as bathymetry LAS( classification code 27): (A) residential
Details
-
File Typepdf
-
Upload Time-
-
Content LanguagesEnglish
-
Upload UserAnonymous/Not logged-in
-
File Pages12 Page
-
File Size-