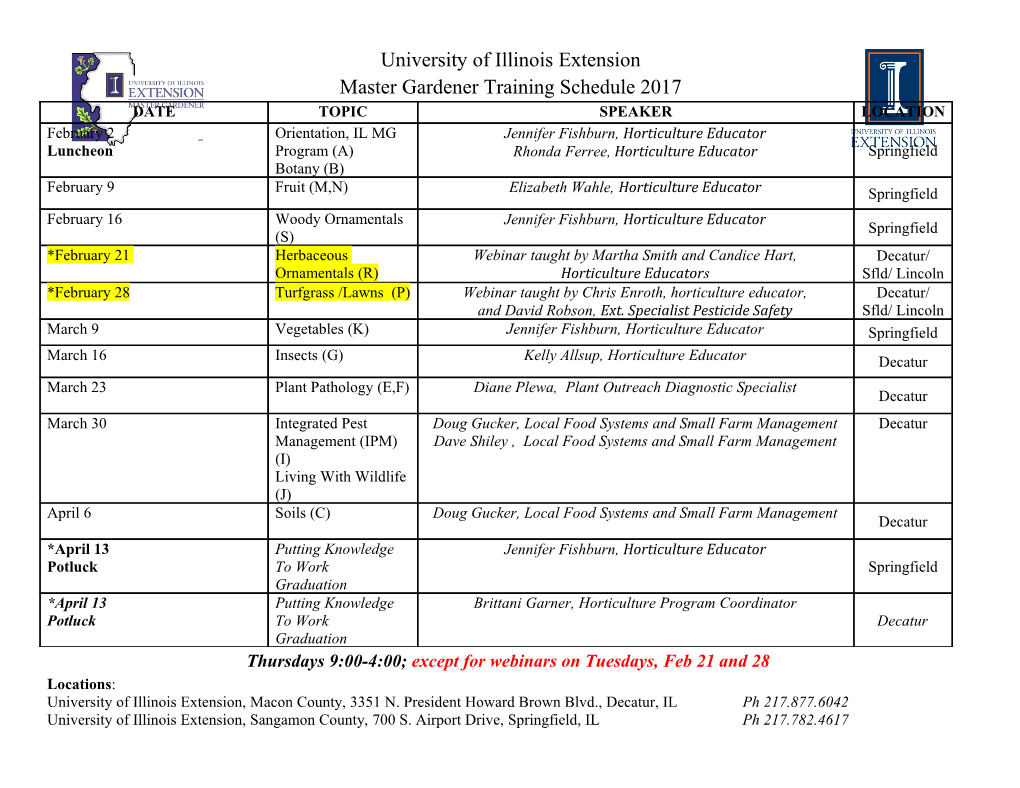
Understanding Defects in Germanium and Silicon for Optoelectronic Energy Conversion by Neil Sunil Patel B.S., Georgia Institute of Technology (2009) Submitted to the Department of Materials Science and Engineering in partial fulfillment of the requirements for the degree of Doctor of Philosophy at the MASSACHUSETTS INSTITUTE OF TECHNOLOGY June 2016 © Massachusetts Institute of Technology 2016. All rights reserved. Author.......................................................................... Department of Materials Science and Engineering May 17, 2016 Certified by . Lionel C. Kimerling Thomas Lord Professor of Materials Science and Engineering Thesis Supervisor Certified by . Anuradha M. Agarwal Principal Research Scientist, Materials Processing Center Thesis Supervisor Acceptedby..................................................................... Donald Sadoway Chairman, Department Committee on Graduate Theses Understanding Defects in Germanium and Silicon for Optoelectronic Energy Conversion by Neil Sunil Patel Submitted to the Department of Materials Science and Engineering on May 17, 2016, in partial fulfillment of the requirements for the degree of Doctor of Philosophy Abstract This thesis explores bulk and interface defects in germanium (Ge) and silicon (Si) with a focus on understanding the impact defect related bandgap states will have on optoelectronic applications. Optoelectronic devices are minority carrier devices and are particularly sensi- tive to defect states which can drastically reduce carrier lifetimes in small concentrations. We performed a study of defect states in Sb-doped germanium by generation of defects via irradiation followed by subsequent characterization of electronic properties via deep- level transient spectroscopy (DLTS). Cobalt-60 gamma rays were used to generate isolated vacancies and interstitials which diffuse and react with impurities in the material toform four defect states (E37,E30,E22, and E21) in the upper half of the bandgap. Irradiations at 77 K and 300 K as well as isothermal anneals were performed to characterize the relationships between the four observable defects. E37 is assigned to the Sb donor-vacancy associate (E- center) and is the only vacancy containing defect giving an estimate of 2 1011 cm 3 Mrad 1 × − − for the uncorrelated vacancy-interstitial pair introduction rate. E37 decays by dissociation 12 3 and vacancy diffusion to a sink present in a concentration of 10 cm− . The remaining three defect states are interstitial associates and transform among one another. Conversion ratios between E22,E21, and E30 indicate that E22 likely contains two interstitials. The formation behavior of E22 after irradiation in liquid nitrogen indicates that E30 is required for formation of E22. Eight defect states previously unseen after gamma irradiation were observed and characterized after irradiation by alpha and neutron sources. Their absence after gamma irradiation indicates that defect formation requires collision cascades. We demonstrate electrically pumped lasing from Ge epitaxially grown on Si. Lasing is observed over a 200 nm bandwidth showing that this system holds promise for low- ∼ cost on-chip communications applications via silicon microphotonics. The observed large threshold currents are determined to be largely a result of recombination due to threading dislocations. We estimate that recombination by threading dislocations becomes negligible when threading dislocation density is . 4 106 cm 2. × − We developed a process for incorporation of colloidal quantum dots (QD) into a chalco- genide glass (ChG) matrix via solution based processing in common solvents. Observation of photoluminescence (PL) comparable to QD/polymethyl methacrylate (PMMA) films shows potential for this material to form the basis for low cost light sources which can be integrated with ChG microphotonic systems. We investigated the impact of surface recombination on the benefit of combining a singlet fission material (tetracene) with a Si solar cell. Our simulations show that for efficiency gains, surface recombination velocity (SRV) for the tetracene/silicon interface must be less 4 1 than 10 cm s− . Characterization via radio frequency photoconductivity decay (RFPCD) measurements show that tetracene does not provide a sufficient level of passivation thus requiring another material which passivates the interface. Using thin films fabricated by atomic layer deposition (ALD), we showed the first direct evidence of triplet energy transfer to Si via magnetic field effect (MFE) PL measurements. Thesis Supervisor: Lionel C. Kimerling Title: Thomas Lord Professor of Materials Science and Engineering Thesis Supervisor: Anuradha M. Agarwal Title: Principal Research Scientist, Materials Processing Center For Mom and Dad 7 Acknowledgments This work would not have been possible without generous support from many people. First and foremost, I must thank my advisors, Prof. Kimerling and Dr. Agarwal, who have taught me more than I could have imagined when I started graduate school. I have become a much better researcher due to their great mentorship. I was also fortunate to work with Dr. Michel who provided valuable guidance and advice for the setup of various experimental apparatuses. Together, Kim, Anu, and Jurgen have formed a fantastic research environment within EMAT. Every EMAT student and postdoc past and present has been a pleasure to work with and I owe all of them thanks. Especially Corentin Monmeyran, who was my partner in studying germanium defects. In addition, the many conversations with Vivek Singh, Rodolfo Camacho-Aguilera, Zhaohong Han, Brian Albert, and Timothy Zens made our office an enjoyable space to work. I’d also like to apologize to Brian Pearson andthe Fitzgerald group for treating their office as a break room. I also want to thank the numerous collaborators I’ve been fortunate enough to work with over the years: Dr. Marco Romagnoli, Prof. Kazumi Wada, Stefano Grillanda, Davide Bianchi, Prof. Kathleen Richardson and her students (David Musgraves, Spencer Novak, Jacklyn Wilkinson), Prof. Clara Dimas, Prof. Moungi Bawendi and his students (Jennifer Scherer, Scott Geyer), and Prof. Marc Baldo and his students (Dan Congreve, Markus Einzinger, Tony Wu). I am also indebted to the many staff members at MIT’s shared facilities, especially Kurt Broderick and Bernard Alamariu. I’ve also benefited greatly from the awe-inspiring DIY skills of David Bono and Dr. Piotr Becla. My time at MIT has been very enjoyable largely due to the support of friends. I especially owe thanks to DMSE classmates Dan Harris, Adam Jandl, Jocelyn Newhouse, Max Solar, and Brian Spatocco for their continued camaraderie. Thanks to Ryan Iutzi for (almost) never saying no to a ski trip. Finally, I want to thank my family for their love and encouragement over the years. This thesis would not exist without the curiosity and confidence they instilled in me. 8 Contents 1 Introduction 21 2 Semiconductor Concepts 25 2.1 Carrier Generation and Recombination..................... 25 2.1.1 Carrier Lifetimes.............................. 25 2.1.2 Generation/Recombination Mechanisms................ 27 2.2 Semiconductor Defects............................... 29 2.2.1 Point Defects................................ 30 2.2.2 Dislocations................................. 32 2.2.3 Surfaces and Interfaces........................... 33 2.3 Dynamic Behavior of Deep Defect States..................... 34 2.3.1 Impact of Defect States on Device Performance............. 37 3 Point Defects in Germanium 39 3.1 Deep Level Transient Spectrocopy (DLTS).................... 39 3.1.1 Theory of Operation............................ 40 3.1.2 Laplace DLTS................................ 43 3.1.3 Determination of Defect Properties.................... 43 3.1.3.1 Free Energy of Ionization.................... 43 3.1.3.2 Defect Concentration...................... 46 3.1.3.3 Real Capture Cross Section................... 48 3.1.4 Experimental Apparatus.......................... 48 3.2 Gamma Irradiation of Germanium........................ 50 3.2.1 Point Defect Production.......................... 50 3.2.1.1 Compton Scattering by 60Co Gamma Irradation....... 51 3.2.1.2 Number of Displacements Generated per Collision..... 52 3.2.2 Experimental Parameters......................... 55 3.2.3 Defect States................................. 55 3.2.4 Defect Reactions.............................. 59 3.2.4.1 Irradiation at 77 K ........................ 59 3.2.4.2 Irradiation at 300 K ....................... 61 3.2.5 Defect Assignments............................ 63 3.2.5.1 Donor-vacancy associate: E37................. 63 3.2.5.2 Interstitial associates: E30, E22 and E21............ 65 10 Contents 3.2.6 Formation of Secondary Defect Associates............... 68 3.2.7 Summary.................................. 69 3.3 Neutron and Alpha Irradiation of Germanium................. 69 3.3.1 Higher Mass Particle Irradiation Sources................ 70 3.3.2 Experimental Parameters......................... 72 3.3.3 Defect States................................. 73 3.4 Future Work..................................... 77 3.4.1 Concentration Dependence on Alpha Dose............... 77 3.4.2 Alpha/Neutron Generated Defect Reactions.............. 77 4 Germanium-on-Silicon Laser 79 4.1 Background..................................... 81 4.2 Ge Laser Fabrication and Design......................... 82 4.2.1 Epitaxial Growth of Ge on Si via UHVCVD............... 83 4.2.2 Germanium n-type Doping.......................
Details
-
File Typepdf
-
Upload Time-
-
Content LanguagesEnglish
-
Upload UserAnonymous/Not logged-in
-
File Pages155 Page
-
File Size-