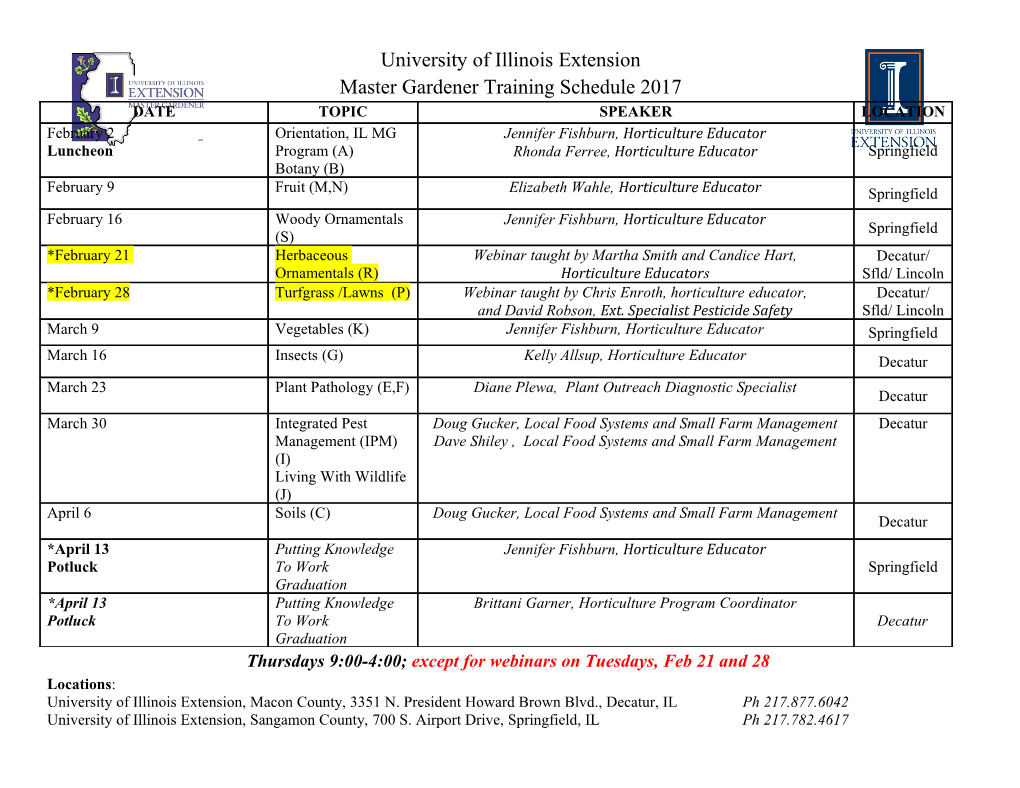
atmosphere Article Mineral Dust and Iron Solubility: Effects of Composition, Particle Size, and Surface Area Aurelie R. Marcotte 1, Ariel D. Anbar 1,2, Brian J. Majestic 3,* and Pierre Herckes 1 1 School of Molecular Sciences, Arizona State University, Tempe, AZ 85287-1604, USA; [email protected] (A.R.M.); [email protected] (A.D.A.); [email protected] (P.H.) 2 School of Earth and Space Exploration, Arizona State University, Tempe, AZ 85287-6004, USA 3 Department of Chemistry and Biochemistry, University of Denver, Denver, CO 80208-9020, USA * Correspondence: [email protected] Received: 23 April 2020; Accepted: 18 May 2020; Published: 21 May 2020 Abstract: There is significant iron deposition in the oceans, approximately 14–16 Tg annually from mineral dust aerosols, but only a small percentage (approx. 3%) of it is soluble and, thus, bioavailable. In this work, we examine the effect of mineralogy, particle size, and surface area on iron solubility in pure mineral phases to simulate atmospheric processing of mineral dust aerosols during transport. Pure iron-bearing minerals common to Saharan dust were partitioned into four size fractions (10–2.5, 2.5–1, 1–0.5, and 0.5–0.25 µm) and extracted into moderately acidic (pH 4.3) and acidic (pH 1.7) leaching media to simulate mineral processing during atmospheric transport. Results show that, in general, pure iron-bearing clay materials present an iron solubility (% dissolved Fe/total Fe in the mineral) an order of magnitude higher than pure iron oxide minerals. The relative solubility of iron in clay particles does not depend on particle size for the ranges examined (0.25–10 µm), while iron in hematite and magnetite shows a trend of increasing solubility with decreasing particle size in the acidic leaching medium. Our results indicate that while mineralogy and aerosol pH have an effect on the solubilization of iron from simulated mineral dust particles, surface processes of the aerosol might also have a role in iron solubilization during transport. The surface area of clay minerals does not change significantly as a function of particle size (10–0.25 µm), while the surface area of iron oxides is strongly size dependent. Overall, these results show how mineralogy and particle size can influence iron solubility in atmospheric dust. Keywords: iron solubility; mineral dust aerosols; particle size; ICP-MS 1. Introduction Oceans are responsible for removing approximately one-third of anthropogenic CO2 from the Earth’s atmosphere [1]. Photosynthetic uptake by marine phytoplankton plays a key role in that removal. In many ocean regions, the rate of photosynthetic uptake is ultimately controlled by upwelling, which transfers nutrients, such as nitrogen, phosphorus, and potassium, to the photic zone from deep waters. However, in some parts of the ocean, the concentration of these nutrients exceeds demand [2]. Iron—which is biologically essential but scarce in upwelled deep ocean water—is the limiting factor in these high nutrient, low chlorophyll (HNLC) regions, which dominate the Southern Ocean [3–6]. There are a variety of iron sources to the open ocean, depending on location. In remote ocean regions, such as the sub-Arctic, Southern Ocean, and equatorial Pacific Ocean, atmospheric mineral dust is a major source of iron, in addition to resuspension of coastal sediments, glacial melt, hydrothermal vents, sediment interactions, and volcanic activity [7–9]. In other ocean regions, atmospheric inputs from urban pollution, specifically oil and coal fly ash, can be a substantial source of iron [10,11]. Atmosphere 2020, 11, 533; doi:10.3390/atmos11050533 www.mdpi.com/journal/atmosphere Atmosphere 2020, 11, 533 2 of 12 Atmospheric mineral dust contains approximately 3% iron by mass and can undergo long range transport, resulting in an estimated 14–16 Tg of iron deposited into the oceans annually [7,12]. However, only a very small fraction of this iron is soluble (~2–4% of iron in clays and ~0.003% from iron (oxyhydr)oxides) [13]. The extent of iron dissolution in dust aerosols is controlled by particle composition (mineralogy), physical and chemical reactions during atmospheric transport, and the presence and mixing of more soluble (10–70%) anthropogenic iron with dust [14–17]. Modelling studies suggest that the long-range transport of aluminosilicate minerals specifically, which release nanoparticulate ferrihydrite and soluble iron, may provide an important source of bioavailable iron to remote ocean regions [18–20]. Insoluble iron in mineral aerosols can dissolve via three different mechanisms: (1) proton-promoted dissolution, where high proton concentrations disrupt Fe-O bonds in the crystal lattice [21,22]; (2) ligand-controlled dissolution, where organic acids form surface complexes with iron and detach to form soluble Fe(III) [23,24]; and (3) reductive dissolution, where Fe(III) forms soluble Fe(II) generally by photochemical reactions [23,25,26]. Although dust-bound iron can be found as both Fe(II) and Fe(III) [27], a majority of iron in dust is found in the oxidized Fe(III) state, which is substituted into aluminosilicate minerals and is present in (oxyhydr)oxides such as goethite and hematite [17,22]. Fe(II)(aq) can be produced by the dissolution of Fe(II) compounds or by the reduction of Fe(III) at the solid/liquid boundary layer [28]. The presence of organic ligands in atmospheric waters, most notably oxalate, increases the concentration of dissolved Fe(II) because these molecules can act as electron donors [29–33]. When included in model calculations, oxalate and photochemical redox cycling increased the dissolved iron fraction delivered to the open ocean by 75% [34]. Furthermore, Myriokefalitakis et al. (2015) found that in present day conditions, approximately 22% of the atmospheric iron dissolution flux is attributed to organic ligand-promoted dissolution [35]. The photochemical reduction of Fe(III) to Fe(II), which then is rapidly reoxidized to Fe(III), is also a major source of Fe(II) [28,29,36,37]. The factors affecting the solubilization of iron during atmospheric transport of mineral dust need to be considered as aerosols are a large source of soluble iron to the open ocean. Three main processes likely to occur during atmospheric transport are: (1) aqueous phase processing (i.e., cloud and marine processing, changes in relative humidity, wetting, and drying cycles), (2) interactions with atmospheric gases (specifically anthropogenic contributions), and (3) photochemistry. Two other factors affecting iron solubilization during transport are related to the composition of the mineral dust: mineralogy, and particle size and surface area. In this work, simulated aqueous phase processing of pure mineral phases is examined as they relate to mineralogy and particle size/surface area. While particle size and surface area have been hypothesized to have a significant impact on iron solubility during transport, few laboratory studies have examined these parameters further [38–41], with most data coming from field measurements. Size is a major factor in how far a mineral dust particle will travel in the atmosphere from its point of origin, and therefore, dictates the quantity deposited in the open ocean [42,43]. Dust particles can range from 0.200 µm to 100 µm in diameter, and particles smaller than 10 µm can be transported long distances, at times hundreds of kilometers, from their source [44]. This study focuses on particles ranging from 10 µm to less than 0.25 µm in diameter with a specific focus on particle sizes between 2.5 µm and 0.25 µm. Particles in this size range are mainly in the accumulation mode, which are most likely to be transported over long distances due to their atmospheric lifetimes and are in the range of particles that will become cloud condensation nuclei and will, therefore, undergo cloud processing [42]. Atmospheric dust typically contains quartz, feldspar, calcite, gypsum, illite, kaolinite, smectite, and hematite [45]. Pure illite, kaolinite, magnetite, hematite, and goethite are examined here as they are considered relevant iron bearing minerals [13,46–48]. Moderately acidic and acidic leaching media were used to examine aqueous atmospheric processing. The moderately acidic leaching medium (pH 4.3) was used to simulate cloud water, while the acidic leaching medium (pH 1.7) was used to simulate a marine aerosol solution. Atmosphere 2020, 11, 533 3 of 12 2. Materials and Methods 2.1. Reagents The moderately acidic leaching media (Table1) was made from chemicals obtained from Sigma-Aldrich including glacial acetic acid (99.8%), sodium acetate (99.0%), formic acid ( 96%), ≥ sodium formate (99+%), and ammonium nitrate (99.999%). The acidic leaching media was prepared with sulfuric acid (10 N, Fisher Scientific, Waltham, MA, USA) and sodium chloride ( 99.5%, Fluka, ≥ Geneva, Switzerland). Table 1. Composition of extraction solutions in solubility experiments. Solution Composition 5 mM acetate buffer *, 5 mM formate buffer **, 5 mM Moderately acidic leaching medium (pH 4.3) ammonium nitrate Acidic leaching medium (pH 1.7) 0.1 M sulfuric acid, 0.1 M sodium chloride * Acetate buffer has a ratio of 0.36 (acetate/acetic acid). ** Formate buffer has a ratio of 3.63 (formate/formic acid). Approximate composition of cloud water [49]. A higher concentration was used in this work (a factor of 10 higher). Approximate composition of an aerosol in marine environments [50,51]. All plasticware was soaked overnight in a 10% nitric acid (OmniTrace, EMD) bath. Mineral extracts were filtered using polyethersulfone (PES) membrane filters (0.22 µm pore size, Millex, Millipore Corporation, Cork, Ireland). For inductively coupled plasma mass spectrometry (ICP-MS) analysis, iron standards were made from a J. T. Baker iron standard (1000 ppm). For UV-Vis analysis, Fe(III) was reduced to Fe(II) with 5.5 mM hydroxylamine hydrochloride (99.999% metals basis, Sigma-Aldrich, St. Louis, MO, USA), and then complexed with 5.2 mM ferrozine solution (3-(2-Pyridyl)-5,6-diphenyl-1,2,4-triazine-4’,4”-disulfonic acid sodium salt, Sigma-Aldrich, St.
Details
-
File Typepdf
-
Upload Time-
-
Content LanguagesEnglish
-
Upload UserAnonymous/Not logged-in
-
File Pages12 Page
-
File Size-