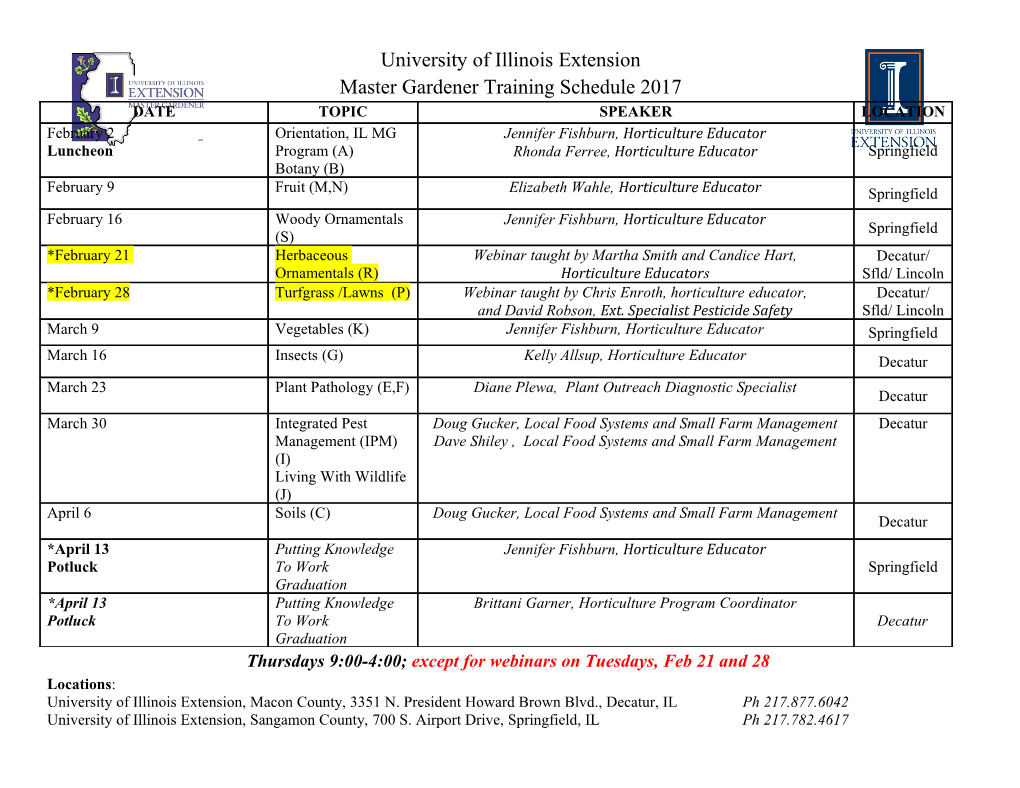
A Study on the Photovoltaic Effect - Solar Cells A Group Project by Thaveesakdi Keowsim, Project Coordinator (Thailand) Jean Baptiste Bwanakeye (Rwanda) Htein Lin (Burma) University of Florida Training in Alternative Energy Technologies Program Sponsored by The U.S. Agency For International Development (US AID) 5th Session (22 February - 4 June 1982) Contents Page Acknowledgement ...... ... ......................... i Proposal .... .... ............................ .. Abstract .... .. ... ............................ .. iv I. Theoretical Studies ........ ....................... 1 A Solar Radiation Principles and Properties ............. .. B. Semiconductor Basics .... .... .................... 2 1. Band Theory ...... .. ....................... 2 2. Optical Effects ....... ..................... 4 3. Photovoltaic Junction Properties ..... ........... 6 a. P-N Junction .... .. ... .................. 6 b. Voltage-Current Relationship ..... ........... 6 c. Conversion Efficiency and Materials .... ........ 6 d. Limitations ........ .................... 8 e. Temperature Effects ..... .. ................ 8 C. The Principles Underlying the Photcvoltaic Effect ........10 1. Light Absorption in Semiconductor ... ............ .. 10 2. Charge Separation in the Photovoltaic Cell ... ....... 10 3. Migration of Charge Carriers to the Charge Separation Site ..... ........................ 11 4. Short Circuit Current ....... .................. 12 5. Current Voltage Characteristic and Maximum Efficiency . 13 6. Open Circuit Voltage and Its Dependence on 10 ......... 17 7. Dependence of rmax on EG .... .. ................ 20 8. Dependence of n max on Temperature ... ........... .. 21 II. Experimental Results ....... ...................... .. 22 Experiment #1 To Investigate a Matched Pair of Silicon Solar Cells ... ............ .. 23 Experiment #2 I-V Characteristics of 5 Silicon Solar Cells in Series .... .. ............... 26 Experiment #3 Variation of V and Isc vs Time, t, of the 5-SolarU~ells in Series ... ....... 33 Experiment #4 Shadow Effect on the Test-Solar Cell .......38 III. The Cost of the System ....... ..................... 40 IV. Conclusion .... .. ... .. .......................... 42 V. References .... .. .... ........................... 44 Acknowledgement We wish to express our sincere thanks to the TAET staff in kindly supporting us with materials, documents, references, advice, laboratory facilities and administrative facilities. Their encouragement and under­ standing make the result ef the completion of this project work possible. We do appreciate the government of the United States of America through the Agency for International Development (AID) in granting us the sponsor­ ship to participate in this program. Proposal A. Objective: 1. Tc study the theoretical basic principles underlying the photovoltaic effect which apply to solar cells in detail. 2. Experiment on solar cells for studying some properties of solar cells: - To investigate a matched pair of solar cells. - I-V characteristics of solar cells. - Variation of the open circuit voltage and short circuit current of solar cell with the time of light-exposure. - Shadow effect on the test cell. B. Design, equipment and components required: 1. Silicon solar cells 2. Solar cell panel 3. Digital multimeter 4. AVO-meter 5. Solar radiometer 6. Load resistor (10 a) 7. Rheostat (16 - 220 si) 8. Watch 9. Artificial sunlight source (photo-lamp) C. Experimentation: 1. To investigate a matched pair of solar cells by measuring Voc and Isc of each cell. 2. To observe the I-V characteristics of solar cells by measuring the load current and load voltage at various values of load resistance. ii 3. To observe the variation of Voc and Isc of solar cells by measuring Voc and Isc vs time. 4. To study the effect of shadow on the test cell by measuring the test cell voltage, load voltage and load current at various shadow condi­ tions on the test cell. D. Application: In our countries where there is no electricity available such as in some isolated remote rural areas, electricity from solar cells or solar cell panels might be economically applicable to serve the following needs: 1. Lighting 2. Water pumping 3. Telecommunication 4. Health Centers 5. Schcols iii Abstract Solar cells and solar cell panels are the current devices of the photovoltaic effect, in which the solar energy can be directly converted into electrical energy. In this report, the theorLtical basic principles underlying the photovoltaic effect are presented in detail. Experiments concerning the basic characteristics of solar cells were conducted in the laboratory with silicon solar cells. Experimental results are presented. The correlation between the theoretical and experimental values were found. iv I. Theoretical Studies The physical principles and radiation - material interactions of the photovoltaic effect are described. A. Solar Radiation Principles and Properties: Electromagnetic radiation emanating from the sun is received by the earth at a rate of 5.1 x 102 1 BTU/yr. It is not surprising, therefore, that major interest exists for the capture and conversion to useful work of a significant fraction of this amount of inexhaustible energy. However, radiative energy such as sunlight is a relatively dilute form and hence requires large collection areas to provide the energy equivalent to that obtained from fossil and nuclear fuels. Further, despite the inexhausti­ bility feature, soiar radiation has the characteristic of intermittency due to: (a) the earth's diurnal cycle, (b) seasonal angular displacement. (c) localized weather condition, e.g., clouds, particulate absorption and scattering, etc. Light is a form of energy and the smallest unit of light energy that can be transferred in a process is called a photon. The energy of the photon is related to the frequency of its associated radiation by the equa­ tion E = hv, where h is equal to Planck's constant, 6.62 x 10-27 erg-sec and v is the radiation frequency. Since the frequency v is inversely propor­ tional to the radiation wavelength, viz., v , where A is the wavelength and c is the velocity of light, it is possible to determine the energy associated with photons of a given wavelength, A. Such energy is often expressed in electron-volts (eV). B. Semiconductor Basics: The role played by semiconductor materials is crucial in the energy conversion process. A brief review of semiconductor theory is given here. 1) Band Theory The conductivities of solid materials vary extensively. Representative values -7 may range from less than 2 x 10 mhos/meter for fused quartz, an 7 insulator, to abuut 6 x 10 mhos/meter for silver, a conductor. Materials with intermediate conductivity values are called semiconductors. The distinguishing features of conductors, insulators, and semiconductors can be explained in terms of what is called the energy band concept of materials. Electrons in isolated atoms can exist only at discrete energy levels and are restricted in number at any allowed energy level. When atoms are brought close together, as in a crystal, so that their potential functions overlap, the energy level must split and form clusters of acceptable energy levels. These clusters, or bands, consist of a large number of closely packed discrete energy levels. There are as many levels in the bands as there are atoms in the crystal and as many bands as there are energy levels in an isolated atom of that material. The energy width of these bands depend on the particular material and its atomic spacing. The electrons are constantly seeking the lower energy levels but can be excited to higher states by interaction with photons. The distribution of electrons in the outermost or highest energy bands determine most uf the electrical and thermal properties of the material. If a crystal, for exam­ ple, of a metal, contains an outermost band which is partially filled, an externally applied electric field can shift the occupation of the energy levels and cause a current to flow. If a band is completely empty, there can be no contribution to an electric current by the band. These materials 2 are then good electrical insulators. The highest occupied band corresponds to the ground state of the valence electrons in the atom. For this reason the upper occupied band is called the valence band. In an insulator, the valence band is full. In addition, the width of the forbidden energy gap between the top of the valence band and the next allowed band, called the conduction band, is so large that under ordinary circumstances a valence electron can accept no energy at all from an applied field. Semiconductors are similar to insula­ tors, except that in semiconductors the forbidden gap is much narrower. In a semiconductor at room temperature, though the valence band is full, some electrons may receive enough energy from light so that they may jump the narrow forbidden energy gap into the empty conduction band. The greater the intensity of the light source, the larger the number of electrons that will be excited across the gap. The electrons that are raised are then free to accept electrical energy from an applied field and to move through the crys­ tal. In addition, the sites or "holes" left vacant in the valence band become charge carriers themselves. An electron near a hole can jump in and fill it, leaving a new hole in the place it has occupied, and this in turn can be filled by d neighbor, and so on. Current is actually carried by electrons but it can also be pictured as a flow of positively charged
Details
-
File Typepdf
-
Upload Time-
-
Content LanguagesEnglish
-
Upload UserAnonymous/Not logged-in
-
File Pages51 Page
-
File Size-