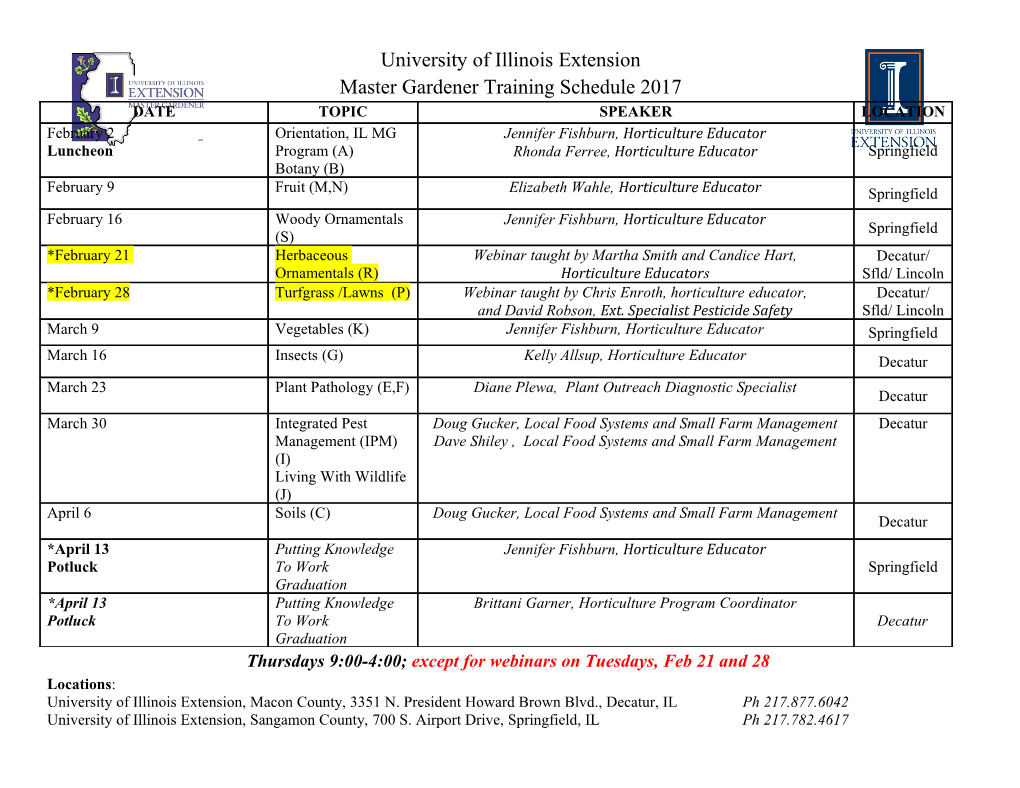
PHYSICAL REVIEW APPLIED 1, 014007 (2014) Coherent Control of Plasmon Propagation in a Nanocircuit Christian Rewitz,1 Gary Razinskas,2 Peter Geisler,2 Enno Krauss,2 Sebastian Goetz,1 Monika Pawłowska,1 † Bert Hecht,2,3,* and Tobias Brixner1,3, 1Institut für Physikalische und Theoretische Chemie, Universität Würzburg, Am Hubland, 97074 Würzburg, Germany 2Nano-Optics and Biophotonics Group, Experimentelle Physik 5, Universität Würzburg, Am Hubland, 97074 Würzburg, Germany 3Röntgen Center for Complex Material Systems (RCCM), Am Hubland, 97074 Würzburg, Germany (Received 12 December 2013; published 27 February 2014) The miniaturization of optical devices is a prerequisite for broadband data-processing technology to compete with cutting-edge nanoelectronic circuits. For these future nano-optical circuits, controlling the spatial and temporal evolution of surface plasmons, i.e., propagating optical near fields at metal-insulator interfaces, is a key feature. Here, we design, optimize, and fabricate a nanoscale directional coupler with one input and two output ports, a device that is an essential element of nano-optical circuits. The directional coupler is based on a two-wire transmission line supporting two plasmonic eigenmodes that can be selectively excited. By manipulating the input polarization of ultrashort pulses and pulse pairs and by characterizing the light emitted from both output ports, we demonstrate open-loop ultrafast spatial and spatiotemporal coherent control of plasmon propagation. Because of the intuitive and optimized design, which exploits a controlled near-field interference mechanism, varying the linear input polarization is enough to switch between both output ports of the nanoscale directional coupler. Since we exploit the interference of a finite spectrum of eigenmodes, our experiments represent a very intuitive classical analogue to quantum control in molecules. DOI: 10.1103/PhysRevApplied.1.014007 Propagating plasmonic modes supported by noble-metal control can rather also be part of the source. First experi- nanowires offer strong subwavelength electromagnetic ments to manipulate propagating plasmons have been energy confinement [1–3] and allow the realization of performed by using networks of chemically grown silver nanometer-scale circuits with well-defined built-in (pas- nanowires [12–17] or slotless gold nanostructures [18]. sive) functionality [4,5] such as splitting or filtering [6]. However, only recent advances in the understanding and Furthermore, (active) coherent control of femtosecond fabrication of plasmonic nanocircuits based on optical optical energy localization in nanoscale random structures antennas [19,20] and two-wire transmission lines [21,22] V and shapes [7] as well as coherent control of plasmon have enabled the quantitative selective excitation of multi- routing has been proposed [8,9]. Coherent control of ple eigenmodes [23]. This possibility prepares the ground nonpropagating near fields was achieved experimentally for an experimental demonstration of coherent control of by using learning algorithms [10,11]. In all these schemes, plasmon propagation based on interference of at least two a far-field laser pulse—determined by its spectral ampli- well-defined modes, similar to concepts used in dielectric tude, phase, and polarization—excites a particular super- waveguides [24]. position of near-field modes, which then evolve into a Here, we report on plasmon routing by open-loop desired state, e.g., a local field enhanced by orders of coherent control in a plasmonic nanoscale directional magnitude [7]. It is customary in the literature [7–9] to call coupler, i.e., a nanocircuit with a single input port and such a scheme “coherent control.” Thus, we will also use two output ports. We show that by careful design of a nano- the term here even when there is no clear separation optical device it is possible to achieve efficient ultrafast between an external “source” for a propagating signal coherent control of highly confined propagating near fields and another field acting as a “control” on that signal. The by using the simplest possible control field, i.e., linearly polarized light, thereby avoiding the use of closed-loop *[email protected]‑wuerzburg.de learning algorithms. In contrast to classical electronic † brixner@phys‑chemie.uni‑wuerzburg.de circuits, where a symmetric bifurcation inevitably causes an equal splitting of any input current pulse towards the Published by the American Physical Society under the terms of the Creative Commons Attribution 3.0 License. Further distri- two output ports, we induce a fundamentally different bution of this work must maintain attribution to the author(s) and behavior in the plasmonic nanoscale directional coupler by the published article’s title, journal citation, and DOI. exploiting the existence of multiple eigenmodes and 2331-7019=14=1(1)=014007(6) 014007-1 Published by the American Physical Society CHRISTIAN REWITZ PHYS. REV. APPLIED 1, 014007 (2014) coherence—both not accessible in classical electronics. We wire after about 2300-nm propagation. We exploit this therefore demonstrate the potential and importance of observation and split the four wires at this point so that optical coherent control in the design and operation of the þ45∘ polarization results in propagation of light in one plasmonic nanocircuitry. branch only. The resulting simulated near-field intensity Our nanoscale directional coupler is based on a two-wire distribution is displayed in Fig. 2(a). Indeed, the intensity is transmission line [21,22] that supports a symmetric [quasi- successfully routed to the right branch and emitted from TM, Fig. 1(a)] and an antisymmetric [quasi-TE, Fig. 1(b)] output port A as shown in Fig. 2(b). Because of symmetry, eigenmode [23,25]. As shown recently [23], pure eigen- output port B lights up for an input polarization of −45∘ modes can be excited with equal amplitude if an appro- [Fig. 2(c)]. For 0° or 90° excitation, the plasmon splits up priate incoupling antenna [20] is attached to the two-wire equally into both branches (see Supplemental Material for transmission line and is illuminated by a well-positioned figures [26]). Beyond the branching region, the modal linearly polarized laser spot. For a focused laser beam composition of the propagating optical near field is domi- positioned on the center of the incoupling antenna, the nated by the antisymmetric mode that is localized in the gap symmetric mode is excited if the polarization is parallel to the transmission line, whereas the antisymmetric mode is and, therefore, radiates to the far field at the point where the launched with perpendicular polarization. Consequently, gap is short cut [23]. The choice of the branching position as for any other polarization state, corresponding superposi- well as the design of the antenna lead to simple rather than tions of both eigenmodes are created. Because of different complex [10,31] optical control fields. λsym ¼ 517 The nanoscale directional coupler was fabricated effective wavelengths of the eigenmodes ( eff nm λantisym ¼ 471 by focused ion-beam milling (FEI company, Helios and eff nm for 800-nm vacuum wavelength), a beating pattern along the propagation direction is formed NanoLab) from a single-crystalline gold flake deposited whose phase, and thereby the position of field maxima and on a cover glass [32] [Fig. 2(d)]. Femtosecond laser pulses minima, can be controlled by the polarization of the and pulse pairs with mutual orthogonal polarization (x and y incoming light. Figure 1(c) shows the S-like near-field polarized) and adjustable time delays were focused onto the ∘ intensity distribution obtained for a þ45 linear polariza- input port of the nanostructure by using a homebuilt inverted tion (see Supplemental Material for details of the numerical confocal scanning microscope [33,34]. The microscope was calculations [26–28]). We note that alternatively the beating also used to image the light emission from the two output pattern can be obtained by considering the two-wire ports of the structure (see Supplemental Material for a transmission line as two (strongly) coupled single-wire detailed description of the measurement scheme [26]). waveguides supporting identical modes [29,30]. To experimentally demonstrate single-pulse routing, we In order to realize a branching region for the two-wire mounted the nanostructure so that the symmetry axis transmission line and achieve phase-sensitive directional coincided with the diagonal of the coordinate system coupling, two additional wires are added on both sides of the spanned by the two orthogonal polarizations. In this two-wire transmission line. These four wires then split up configuration, an x (y)-polarized pulse is expected to excite adiabatically into a set of two uncoupled parallel two-wire the superposition of eigenmodes that leads to far-field transmission lines supporting the same eigenmodes as the emission only at output port A (B) [Figs. 3(a) and 3(b)]. input line. Figure 1(d) illustrates the principle of directional coherent control in this coupler. We follow the evolution of the beating pattern into the four-wire region. For þ45∘ input polarization, an intensity maximum appears at the lowest B (a)A 5 B (b)A B (c)A (d) 5 4 (a) 0.2 0.3 4 air 0 R glass 0 (c) 1000 nm 0 3 I/I I/I I/I (b) I/I 3 -1 -3 10 125 nm 0.0 (d) 0.0 2 10 2 FIG. 1 (color online). Simulated time-averaged near-field in- 1 tensity distributions. (a),(b) Modal profiles of an infinitely 1 long two-wire transmission line situated on a glass substrate. 0 0 500 nm (a) Symmetric and (b) antisymmetric eigenmode. “þ” and “−” indicate the instantaneous charge distribution, and ⊗ and ↔ the FIG. 2 (color online). Nanoscale directional coupler. polarization required to launch the mode. (c) The beating pattern (a) Simulated steady-state near-field intensity distribution in a produced by an equal-amplitude superposition of symmetric and plane 10 nm below the glass-air interface for excitation with þ45∘ antisymmetric modes. The white arrow indicates the incoupling input polarization (white arrow) demonstrating routing to the right polarization.
Details
-
File Typepdf
-
Upload Time-
-
Content LanguagesEnglish
-
Upload UserAnonymous/Not logged-in
-
File Pages6 Page
-
File Size-