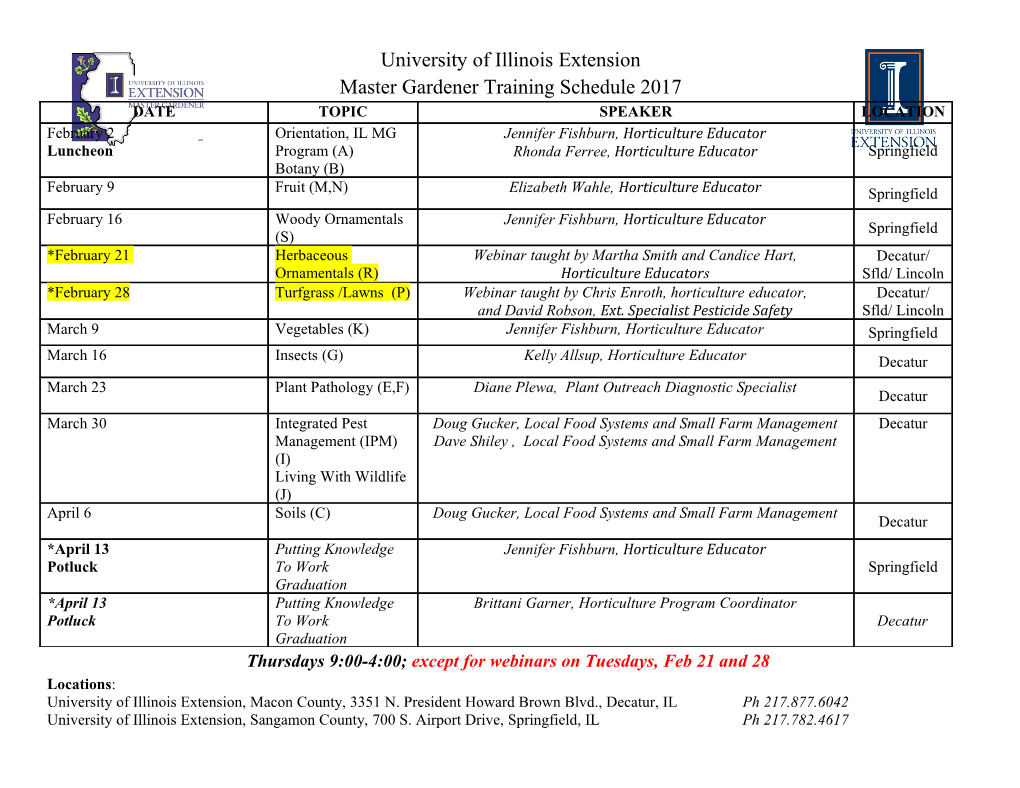
Metabolic Engineering 31 (2015) 44–52 Contents lists available at ScienceDirect Metabolic Engineering journal homepage: www.elsevier.com/locate/ymben Consolidated bioprocessing of cellulose to isobutanol using Clostridium thermocellum Paul P. Lin a,1, Lou Mi a,1, Amy H. Morioka a, Kouki M. Yoshino a, Sawako Konishi a, Sharon C. Xu a, Beth A. Papanek c,d, Lauren A. Riley c,e, Adam M. Guss c,d,e, James C. Liao a,b,n a Department of Chemical and Biomolecular Engineering, University of California, Los Angeles, CA 90095, USA b UCLA-DOE Institute of Genomics and Proteomics, USA c Biosciences Division, Oak Ridge National Laboratory, Oak Ridge, TN 37831, USA d Bredesen Center for Interdisciplinary Research and Graduate Education, University of Tennessee, Knoxville, TN 37996, USA e BioEnergy Science Center, Oak Ridge National Laboratory, Oak Ridge, TN 37831, USA article info abstract Article history: Consolidated bioprocessing (CBP) has the potential to reduce biofuel or biochemical production costs by Received 28 April 2015 processing cellulose hydrolysis and fermentation simultaneously without the addition of pre- Received in revised form manufactured cellulases. In particular, Clostridium thermocellum is a promising thermophilic CBP host 6 June 2015 because of its high cellulose decomposition rate. Here we report the engineering of C. thermocellum to Accepted 1 July 2015 produce isobutanol. Metabolic engineering for isobutanol production in C. thermocellum is hampered by Available online 10 July 2015 enzyme toxicity during cloning, time-consuming pathway engineering procedures, and slow turnaround Keywords: in production tests. In this work, we first cloned essential isobutanol pathway genes under different Biofuel promoters to create various plasmid constructs in Escherichia coli. Then, these constructs were Consolidated bioprocessing transformed and tested in C. thermocellum. Among these engineered strains, the best isobutanol Clostridium thermocellum producer was selected and the production conditions were optimized. We confirmed the expression Butanol of the overexpressed genes by their mRNA quantities. We also determined that both the native ketoisovalerate oxidoreductase (KOR) and the heterologous ketoisovalerate decarboxylase (KIVD) expressed were responsible for isobutanol production. We further found that the plasmid was integrated into the chromosome by single crossover. The resulting strain was stable without antibiotic selection pressure. This strain produced 5.4 g/L of isobutanol from cellulose in minimal medium at 50 oC within 75 h, corresponding to 41% of theoretical yield. & 2015 International Metabolic Engineering Society. Published by Elsevier Inc. All rights reserved. 1. Introduction rate. Recent studies of metabolic features of C. thermocellum (Zhou et al., 2013) and advances in genetic modification tools (Tyurin Lignocellulose instead of sugar as the raw material for biofuel et al., 2004; Tripathi et al., 2010; Argyros et al., 2011) for C. and biochemicals production can potentially provide the quantity thermocellum make the CBP organism an attractive platform for needed to make a significant impact, improve net carbon and biofuel or biochemical production. energy balances, lower production cost, and avoid the food vs. fuel Longer-chain alcohols offer advantages as a gasoline substitute dilemma (Lynd et al., 2005, 2008). However, biomass recalcitrance or drop-in fuel (Atsumi et al., 2008). In particular, isobutanol —resistance to degradation—currently limits the use of lignocellu- received significant attention because it can be used as fuel or a lose. Consolidated bioprocessing (CBP) is a potential solution in feedstock chemical. Isobutanol can be dehydrated to form iso- which cellulose hydrolysis and fermentation occur simultaneously butene, which can then be oligomerized to C8 then C12 alkenes to without added cellulase. Clostridium thermocellum is a promising be use as jet fuel. The C8 alkene can also be dehydrocyclized to thermophilic CBP host because of its high cellulose deconstruction form p-xylene Peters et al., (2011), which can then be oxidized to form terephthalic acid as a monomer for the common plastic polyethylene terephthalate (PET). Microbial production of isobu- n Corresponding author at: Department of Chemical and Biomolecular Engineer- tanol from renewable sources has been demonstrated in multiple ing, University of California, Los Angeles, 5531 Boelter Hall, 420 Westwood Plaza, engineered organisms (Atsumi et al., 2008, 2009; Smith et al., Los Angeles, CA 90095, USA. Fax: þ1 310 206 4107. E-mail address: [email protected] (J.C. Liao). 2010; Higashide et al., 2011; Li et al., 2012; Lin et al., 2014), 1 These authors contributed equally to the work. indicating the flexibility of the pathway. Isobutanol production http://dx.doi.org/10.1016/j.ymben.2015.07.001 1096-7176/& 2015 International Metabolic Engineering Society. Published by Elsevier Inc. All rights reserved. P.P. Lin et al. / Metabolic Engineering 31 (2015) 44–52 45 from cellulose has also been demonstrated using a cellulolytic Madison, WI) were used as host for plasmid construction. Strains organism, Clostridium cellulolyticum (Higashide et al., 2011). How- and plasmids used in this study are listed in Table 1. ever, this organism has a low cellulolytic rate and a long doubling All plasmids were constructed by DNA assembly techniques. time, and is not suitable for CBP . C. thermocellum offers much Both vector and inserts (target genes) were amplified by PCR using higher cellulose decomposition rate and has the ability to grow at Phire Hot Start II DNA polymerase (Thermo Scientific, Hudson, elevated temperatures (50–60 oC), which facilitate cellulose degra- NH). PCR products were purified by a PCR purification Kit (Zymo dation and reduce the chance of contamination compared to the Research, Irvine, CA). The vector and insert were mixed with case at mesophilic temperatures. Here, we seek to produce Gibson Assembly Master Mix (New England Biolabs, Ipswich, MA) isobutanol directly from cellulose to achieve high titer using C. and incubated at 50 1C for 1 h. Then the assembly product was thermocellum. transformed to BL21 or MDS™42 LowMut ΔrecA strain. The C. thermocellum genetic tools (Tripathi et al., 2010; Argyros presence of correctly cloned inserts was determined by colony et al., 2011; Guss et al., 2012; Deng et al., 2013) and isobutanol PCR and DNA sequencing (Retrogen, San Diego, CA). pathway at elevated temperatures (Lin et al., 2014) have been previously reported. In addition, selected C. thermocellum promo- 2.2. Chemicals and reagents ters have been characterized (Olson et al., 2015). However, the apparent toxicity of the isobutanol pathway genes severely limits All chemicals unless otherwise specified were acquired from the applicability of these genetic systems. Thus, we developed a Sigma-Aldrich (St. Louis, MO) or Thermo Scientific. Phire Hot Start strategy to overcome this problem and screened for appropriate II DNA polymerase was purchased from New England Biolabs. promoter combinations to express the necessary genes for the pathway, and constructed various strains for isobutanol produc- 2.3. Media and cultivation tion using the available plasmid-based system (Argyros et al., 2011; Guss et al., 2012; Deng et al., 2013). We then characterized All E. coli strains were grown in LB or TB medium containing the strains constructed, determined the gene copy number, iden- appropriate antibiotics at 37 1C on a rotary shaker (250 rpm). tified native enzymes potentially involved in isobutanol biosynth- Antibiotics were used at the following concentrations: ampicillin, esis, and optimized the production conditions. 200 μg/ml; kanamycin, 50 μg/ml; chloramphenicol, 20 μg/ml. Except for small scale isobutanol production, all C. thermocel- lum strains were cultured inside a Coy anaerobic chamber (Coy Laboratory Products, Grass Lake, MI) in a modified CTFuD medium 2. Methods (Tripathi et al., 2010)at501C incubation . CTFuD medium contains the following components: 3 g/L of sodium citrate tribasic dehy- 2.1. Bacterial strains and plasmids drate, 1.3 g/L ammonium sulfate, 1.43 g/L potassium phosphate monobasic, 1.37 g/L potassium phosphate dibasic, 0.5 g/L cysteine- C. thermocellum DSM 1313 Δhpt was a gift from Katherine Chou HCl, 21 g/L MOPS, 6 g/L glycerol-2-phospate disodium, 5 g/L cello- from the National Renewable Energy Laboratory. We referred C. biose, 4.5 g/L yeast extract, 0.01 g/L calcium chloride, 0.011 g/L thermocellum DSM 1313 Δhpt as the wild type strain in this study magnesium chloride, 0.0006 g/L ferrous sulfate heptahydrate, because the Δhpt is used for the sole purpose of counter-selection 0.01 g/L thiamin, and 0.001 g/L resazurin. Antibiotics were used when needed, and has no effect on growth and fermentation. at the following concentrations: thiamphenicol 20 ug/ml. In addi- Escherichia coli BL21 (New England Biolabs, Ipswich, MA) and tion, 2.5 g/L sodium bicarbonate was used to enhance C. thermo- MDS™42 LowMut ΔrecA (Pósfai et al., 2006) (SCARAB genomics, cellum growth. Table 1 List of strains and plasmids used in this study. Name Descriptiona Reference Strain E. coli BL21 fhuA2 [lon] ompT gal [dcm] ΔhsdS New England Biolabs MDS™42 MG1655 multiple-deletion strain (1) ΔdinB ΔpolB ΔumuDC (2) ΔIS609 ΔpatD ΔydcV ΔydcU ΔydcT ΔydcS ΔydcR ΔhicA ΔhicB SCARAB genomics LowMut ΔyncJ ΔydcP ΔydcN ΔydcO ΔydcM ΔrecA (1819) The recA 1819 mutation is a deletion of recA ΔrecA C. thermocellum DSM 1313T Δhptb National Renewable Δhpt Energy Laboratoryc
Details
-
File Typepdf
-
Upload Time-
-
Content LanguagesEnglish
-
Upload UserAnonymous/Not logged-in
-
File Pages9 Page
-
File Size-