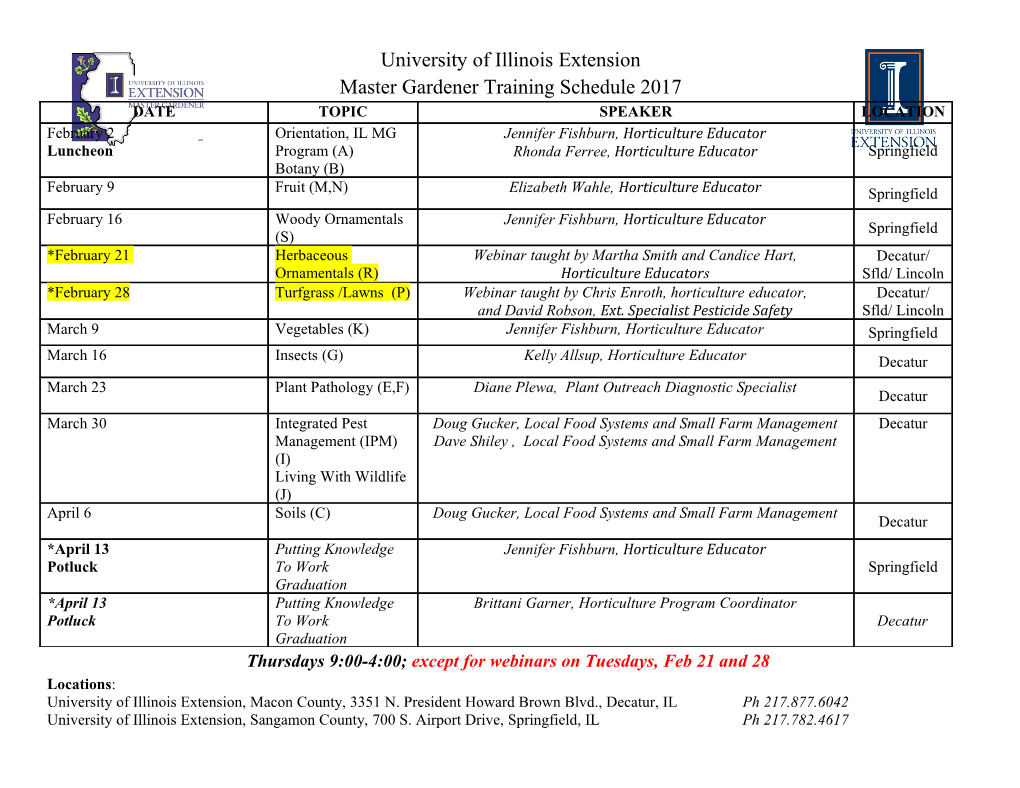
EXPLORING THE BIOFLUIDDYNAMICS OF SWIMMING AND FLIGHT David Lentink Promotor prof. dr. ir. Johan L. van Leeuwen Hoogleraar in de Experimentele Zoölogie, Wageningen Universiteit. Copromotoren prof. dr. Michael H. Dickinson Esther M. and Abe M. Zarem Professor of Bioengineering, California Institute of Technology, United States of America. prof. dr. ir. GertJan F. van Heijst Hoogleraar in de Transportfysica, Technische Universiteit Eindhoven. Overige leden prof. dr. Peter Aerts promotiecommissie University of Antwerp, Belgium. prof. dr. Th omas L. Daniel University of Washington, United States of America. prof. dr. Anders Hedenström Lund University, Sweden. prof. dr. Jaap Molenaar Wageningen Universiteit. EXPLORING THE BIOFLUIDDYNAMICS OF SWIMMING AND FLIGHT David Lentink Proefschrift ter verkrijging van de graad van doctor op gezag van de rector magnifi cus van Wageningen Universiteit, prof. dr. M.J. Kropff in het openbaar te verdedigen op dinsdag 9 september 2008 des namiddags te vier uur in de Aula. Lentink, D. (2008) Exploring the biofl uiddynamics of swimming and fl ight. PhD thesis, Experimental Zoology Group, Wageningen University. P.O. Box 338, 6700 AH Wageningen, the Netherlands. Subject headings: biofl uiddynamics/vortex/stability/chaos/scaling/swimming/fl ight/design. ISBN 978-90-8504-971-5 Summary any organisms must move through water or air in order to survive and reproduce. Th erefore Mboth the development of these individuals and the evolution of their species are shaped by the physical interaction between organism and surrounding fl uid. One characteristic of macro- scopic animals moving at typical speeds is the appearance of vortices, or distinct whorls of fl uid. Th ese vortices are created close to the body as it is driven by the action of muscles or gravity, then are ‘shed’ to form a wake (in eff ect a trackway left behind in the fl uid), and ultimately are dissipated as heat. It is useful to analyze fl uid motion as a collection of vortices, yet the dynamics are complex: vortices interact with the moving organism, interact with each other, and evolve independently in time. Th is research examines two fl ow phenomena that are central to the locomotory perfor- mance of certain organisms. Th e fi rst of these is leading-edge vortex stability. A tornado-shaped vortex has been observed above insect wings, parallel to the leading edge. Th e leading edge vortex substantially augments lift and is integral to insect fl ight. Here I consider in detail the conditions that stabilize this fl ow pattern. Th e second dynamical phenomenon is vortex wake periodicity. Depending on conditions, the wake structure behind a moving organism can be regular and predictable, or chaotic. Although the fl uid fl ow is always deterministic, in the latter case its exact structure becomes hypersensitive to small disturbances. Th is can reduce the ‘forecast horizon’ within which fl uiddynamic forces acting on the body can be reliably predicted. Here I describe the onset of chaotic vortex interactions in biologically relevant models, and consider the conse- quences for feedback-mediated neural control. Th ese studies were carried out using models that represent swimming fi sh, fl ying insects, autorotating plant seeds, and birds. Th e fl ow patterns and forces were observed using (in order of increasing realism) a two-dimensional fl apping foil in a soap fi lm tunnel, a dynamically scaled three-dimensional robotic fl y and seed wing in oil, and freeze-dried swift wings in a wind tunnel. Th e measurements were designed and understood by means of dimensional analysis: dimensionless parameters can identify the fl uid accelerations and stresses that dominate the fl ow; when mapped as a function of morphological and kinematic variables that produce the fl ow, they yield an overview of the biofl uid-dynamical parameter space. Using this framework we were able to show that: (1) Symmetric and periodic fl apping fi ns and wings can produce asymmetric and chaotic vortex wakes. (2) Rotational accelerations stabilize leading edge vortices on revolving wings of insects and other organisms. (3) Stable leading edge vortices augment lift in both animal and plant fl ight. (4) Wing morphing in birds drastically improves glide perfor- mance. (5) Flapping insect wings are less effi cient than spinning and translating insect wings. Th is reverse-engineering analysis of biofl uidic locomotion has furthermore helped us to forward-engineer two micro-air vehicles. We have designed, built, and fl own a robotic fl apper (DelFly) and a morphing model swift (RoboSwift). Clearly the formal methods and fi ndings presented here can lead directly to novel technological products. Exploring the biofl uiddynamics of swimming and fl ight 1.1 Exploring the biofl uiddynamics of swimming and fl ight <3> 2 Designing a map for exploration 3.1 Vortex-wake interactions of a fl apping foil that models swimming and fl ight <43> 3.2 Wake visualization of a heaving and pitching foil in a soapfi lm <57> 3.3 Symmetrically and periodically fl apping foils mediate chaotic vortex-wake interactions <71> 4 Take-off into the third dimension 5.1 A leading edge vortex prolongs descent of maple seeds <111> 6 Escape into thin air 7.1 Biofl uiddynamics as an inspiration for design <145> 8 Back to the future 9.1 Affi liation of co-authors <169> 9.2 Acknowledgements <171> 9.3 Curriculum Vitae <175> As an inspiration for design Introduction 1 2.1 Biofl uiddynamic scaling of fl apping, spinning and translating fi ns and wings <15> A Journey through fl atland 3 4.1 Rotational accelerations stabilize leading edge vortices on revolving fl y wings <81> Swirling down to earth 5 6.1 Turning on a dime <125> 6.2 How swifts control their glide performance with morphing wings <129> Synthesis 7 8.1 Quantifying the development of zebra fi sh swimming performance <161> 9.4 Publication list <177> 9.5 WIAS TSP <179> 9.6 Photo credit <181> 9.7 Samenvatting <183> 1 Introduction 1.1 EXPLORING THE BIOFLUIDDYNAMICS OF SWIMMING AND FLIGHT David Lentink Adaptations for locomotion e are surrounded by organisms that seem to walk, swim and fl y eff ortlessly while traveling Wlong distances. In fact, we are profi cient long distance runners ourselves and adaptations like sweating help us to stay cool. Presumably we can run marathons today because our pred- ecessors used to catch prey on the African savannah by chasing them down for hours, until the prey was exhausted and could be caught easily (Bramble and Lieberman, 2004). Even these days, some of us enjoy chasing down animals. Such as Huw Lobb, who is the fi rst man ever to beat a horse in the annual ‘man against horse race’ of 2004 over 22 miles at Llanwrtyd Wells in mid Wales. While it was a triumph for Huw, who won one of the biggest unclaimed prizes in British athletics of £25,000, Bookie William Hill had to pay out on scores of bets struck at odds of 16/1 (BBC News, 13-06-2004). Th is illustrates how we are fascinated with the locomotory capabilities of other organisms, and that we are willing to pay the price just to outperform them. Perhaps we especially admire organisms that swim and fl y, for which we are poorly adapted. Most of us have learned about our poor adaptations for swimming and fl ight as kids, when trying to swim in deep water and falling from heights. How can we better understand the adaptations needed for swimming and fl ying? Swimming and fl ight is constrained by fl uid dynamics Th e habitats on earth can be divided in land, water, air and their interfaces. Th e physical proper- ties of these habitats constrain the locomotion of organisms. Just think of us running; we not only depend on friction to push off ; the deformational properties of the material on which we run directly aff ects running effi ciency. We experience these diff erent effi ciencies when running on streets consisting of solid materials versus sand, which is a granular material that shares mate- rial properties of both solids and fl uids. Understanding the diff erences in dynamical properties between solids and fl uids gives insight in how these properties can constrain locomotion. Fluids behave very diff erently compared with solid and granular materials, they cannot resist a load without deforming continuously. We experience this diff erence in material properties of land versus water when we attempt to walk over water and sink straight to the bottom. When we swim back to land, we are moving packets of water backwards with our hands, arms and legs. Th ese packets of water resist being moved, which enables us to push off against these packets and swim forward. Hence we rely on the dynamic properties of water to move through it. From a physical point of view, the dynamics of water and air are similar irrespective of their diff erent material properties, such as density and viscosity. Th ey are both fl uids because they only resist rate of deformation, but not deformation itself. Th is particular dynamical property of fl uids is captured by the Navier-Stokes equation, which describes the dynamics of fl uid motion. Compared to our 1.1 Exploring the biofl uiddynamics of swimming and fl ight 4 humble swimming performance and our inability to fl y, many organisms have evolved into spe- cialists with adapted morphology and body kinematics tuned to match the dynamical properties of fl uids, which enables them to swim and fl y well. E.g. half of all vertebrates are fi sh, moving through water, roughly a quarter of all mammals are bats fl ying through the air, and the majority of insects and birds can fl y.
Details
-
File Typepdf
-
Upload Time-
-
Content LanguagesEnglish
-
Upload UserAnonymous/Not logged-in
-
File Pages192 Page
-
File Size-