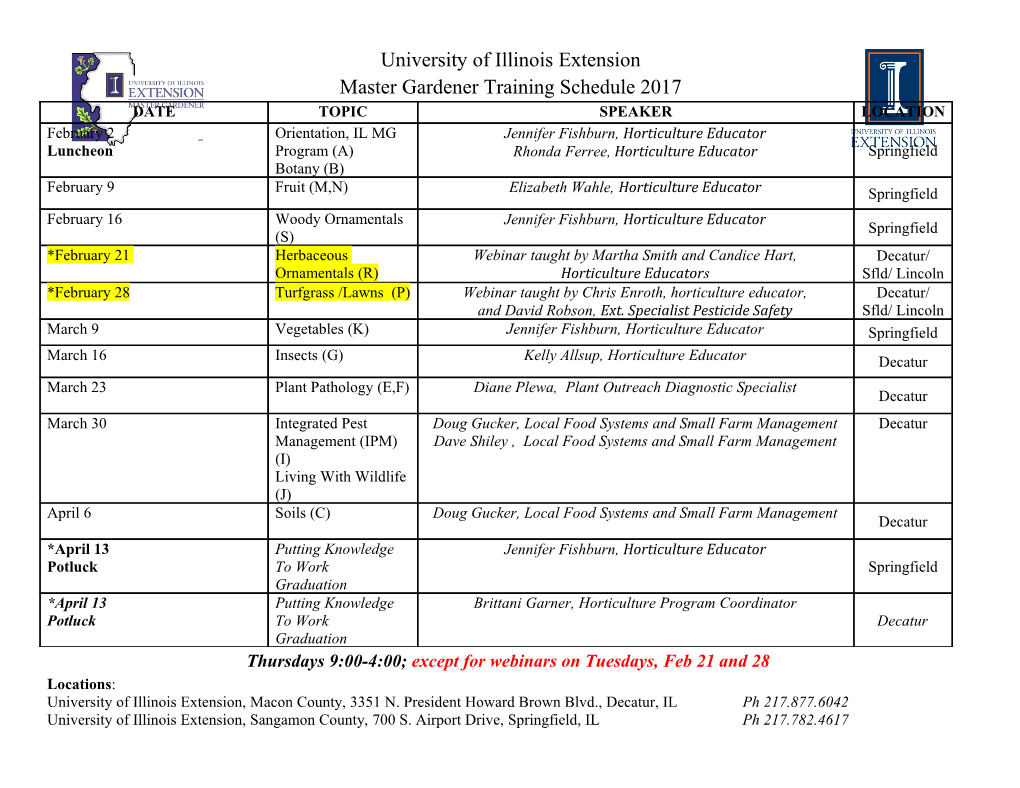
Aeroelasticity Lecture 5: Practical Aircraft Aeroelasticity G. Dimitriadis 1 Introduction • In the previous lecture we showed that Theodorsen theory can be used to obtain an algebraic system of two equations for the two unknowns: – Flutter frequency – Flutter airspeed 2 Non-sinusoidal motion • This algebraic system of equations is only valid if the system is undergoing purely sinusoidal oscillations. There are three situations in which this can happen: – At the flutter condition – At wind-off conditions if the structural damping is zero. – At any airspeed if the wind response to an external sinusoidal load. • The last situation means that we can calculate the response of an aeroelastic system with Theodorsen aerodynamics to any excitation force. • This is particularly useful because it will allow us to calculate the natural frequencies and damping ratios at all airspeeds, as we did with time-domain analysis. 3 Forced response • Until now we have only considered unforced motion: ! $! $ ! $! $ ! $ m S h!! Kh 0 h −l(t) # &# &+# &# & = # & # S I &# & # 0 K & # m(t) & " α %" α!! % " α %" α % " % • No we will consider an external loads (e.g. control input) applied on one of the degrees of freedom. E.g. force on plunge: ! $! $ ! $! $ ! $ ! $ m S h!! Kh 0 h l(t) Fh t # &# &+# &# &+# & = # ( ) & # S I &# & # 0 K & # m(t) & # & " α %" α!! % " α %" α % " − % " 0 % • Or moment on pitch: ! $! $ ! $! $ ! $ ! $ m S h!! Kh 0 h l(t) 0 # &# &+# &# &+# & = # & # S I &# & # 0 K & # m(t) & # M t & " α %" α!! % " α %" α % " − % " α ( ) % 4 Sinusoidal force • If Theodorsen analysis is to be applied, the force must be sinusoidal, e.g. jωt Fh = F0e jωt Mα = M 0e • The steady-state response of the pitch-plunge wing will also be sinusoidal once all the transients have died out, i.e. jωt α = α0e jωt h = h0e • Substituting into the complete equations of motion: 5 Forced equations of motion • Force in plunge " % 2 2 2 " c % 2 $ 2 −ω S + ρπb Ujω + ρπb $x f − 'ω ' $ Kh −ω m + πρUcC(k) jω # 2 & ' $ 2 " % ' $ −ω ρπb " 3 % ' +πρUcC(k)$U +$ c − x f ' jω' $ # # 4 & & ' $ ' " % " h % " % $ 2 3 2 ' 0 F0 Kα −ω Iα +$ c − x f 'ρπb Ujω $ ' = $ ' $ # 4 & '$ α ' $ 0 ' $ −ω 2S − πρUecC(k) jω '# 0 & # & $ " " 3 % % ' −πρUecC k U +$ c − x f ' jω $ " c % 2 2 ( )$ ' ' +$x f − 'ρπb ω # # 4 & & $ # 2 & ' 2 4 $ " c % 2 2 ρπb 2 ' $ −$x f − ' ρπb ω − ω ' # # 2 & 8 & With k=ωb/U 6 FRF Matrix • This equation is of the form H(ω)q0=F • where H-1(ω) is the Frequency Response Function Matrix. • Note that, since H is is a function of ω, the response amplitude q0 will also be a function of ω. • The response is still sinusoidal. But what happens if F is also a function of ω? 7 Fourier Transforms • Using Fourier analysis, any force time signal Fh(t) can be transformed to the frequency domain and written as: jwt F0(ω)e • The signal that is of particular interest here is the impulse. Its Fourier Transform is F0(ω)=1 • This means that if we set F0(ω)=1 and then calculate -1 q0(ω)=H (ω)F • the resulting response amplitude q0(ω), will be the Frequency Response Function (FRF). 8 FRF for pitch-plunge system 9 !'!&( " FRF of h 17.18 & ! 5 & ! 617.18 !'!& & ! 5 The two modes ! ! 632 are clearly present !'!!( :8;<+1=>132 !& !" ! ! " # $ % &! ! " # $ % &! )*+,-+./012345 )*+,-+./012345 !'( !'!# FRF of α " ! 17.1 & " !!'( !'!9 ! 5 ! 617.1 & !& ! The first mode is 5 ! !'!" !&'( present as an anti- 632 !" !'!& :8;<+1=>132 resonance !"'( !9 ! ! " # $ % &! ! " # $ % &! )*+,-+./012345 )*+,-+./012345 9 Impulse Response Function • The Impulse Response Function (IRF) is the inverse Fourier Transform of the FRF. • As we have calculated the FRF, q0(ω), we can simply apply the inverse Fourier Transform to it and calculate the IRF. • The IRF is a time domain signal and it is not sinusoidal. For a dynamic system, the IRF is typically an exponentially decaying sinusoid. • For a fluttering aeroelastic system, the IRF is an exponentially increasing sinusoid. 10 Impulse response of pitch-plunge airfoil !) !# '(&! 9:&),;/<('=;>:!?# )*&! :;"(-<0=*)><?;!'# # &'( " & !'( ! ! !" !!'( 1,234/-(5-/267/-(+7(8 !# 2-3450.*6.03780.*,8*9 !& !$ !&'( ! " # $ % &! &" &# &$ &% ! " # $ % &! &" &# &$ &% *+,-(./0 +,-.*/01 !# !# '(&! )*&! " ! ! " ! ! 1,234/-(5-/267/-(+7( 2-3450.*6.03780.*,8* !" !" ! " # $ % &! &" &# &$ &% ! " # $ % &! &" &# &$ &% *+,-(./0 +,-.*/01 V=15m/s V=25m/s 11 Damped sinusoidal motion • The previous discussion shows that: – Theodorsen aerodynamics is only valid for sinusoidal motion. – Yet Theodorsen aerodynamics can also be used to calculate impulse responses. – The form of the impulse response can tell is if the system is stable or unstable. • Stability analysis is slow and can be less accurate when performed on impulse responses. • We need a method for calculating the damping at all airspeeds directly from the equations of motion. 12 The p-k Method • The p-k method is the most popular technique for obtaining aeroelastic solutions from Theodorsen-type aeroelastic systems • It was proposed in the 80s and since then has become the industrial standard • Virtually all aircraft flying today have been designed using the p-k method 13 Basics • The p-k method uses the structural equations of motion in the standard form ! $! $ ! $! $ ! $ m S h!! Kh 0 h −l(t) # &# &+# &# & = # & # S I &# & # 0 K & # m(t) & " α %" α!! % " α %" α % " % • Coupled with Theodorsen aerodynamic forces of the form With k=ωb/U 14 Time-Frequency domain • The equations of motion have coefficients that depend on the frequency. They are known as time-frequency domain equations. • The complete equations are ! $ 2 ! 3 $ 2 2 # −4πC(k) jk + 2πk −2πcC(k) − 2πbjk − 4πC(k)# c − x f & jk − 2πb k & # " 4 % & ! $! $ ! K 0 $! $ # ! 3 $ &! $ ! $ m S h!! h h 1 2 # 2 & h 0 # &# &+# &# &− ρU 2πec C(k) − 2# c − x f &πbjk # & = # & # S I &# & # 0 K & 2 # " 4 % & 0 " α %" α!! % " α %" α % ! c $ 2 " α % " % # 4πecC(k) jk − 2π #x f − &k 2 & " 2 % ! 3 $ ! c $ b2 # 4 ecC k c x jk 2 x k 2 k 2 & # + π ( )# − f & + π # f − & + π & " " 4 % " 2 % 4 % • and their general form is 1 2 Msq!! + Ksq − ρU Q(k)q = 0 2 15 Basics • Solving time-frequency equations of motion is a nonlinear eigenvalue problem. • The basis of the p-k method is to define d p ≡ • so that dt q!! = p2q • We then re-write the equations of motion as " 2 1 2 % $ p Ms + Ks − ρU Q(k)'q = 0 # 2 & 16 Eigenvalue estimation • These equations of motion are clearly an eigenvalue problem. For a non-trivial solution: " 1 % 2 −1 2 (1) p I + Ms $Ks − ρU Q(k)' = 0 # 2 & • This is one equation with two unknowns, we need a second condition. • Note that p2 is an eigenvalue of the matrix −1 " 1 2 % - Ms $Ks − ρU Q(k)' # 2 & 17 Eigenvalue estimation (2) • But k is a frequency: k=ωb/U. • Recall that the imaginary part of the eigenvalue is the frequency. • Therefore, our second equation is: � �� � = � � (2) • The problem consists of finding an eigenvalue and a frequency that satisfy both equations (1) and (2). 18 The p-k solution • The solution of these equations is iterative. • We guess a value for the frequency k and then we calculate p from the resulting eigenvalue problem (1). • The values of p and k should satisfy equation (2). • If they do not, we change the value of k and re-calculate p until equation (2) is satisfied. • This is called frequency matching. 19 Frequency matching 1. 20 p-k method characteristics • Converges very quickly to the correct eigenvalue. • Suitable for large computational problems. • Calculates complete eigenvalues and therefore damping ratios and natural frequencies. • Valid at all airspeeds, not just the flutter speed. • Estimated flutter speeds are very similar to those obtained from the flutter determinant solution. 21 Sample result 22 Roger’s Approximation • Another way to solve the p-k equations is to transform them completely to the time domain using Roger’s Approximation. • The frequency-dependent part of equations of motion, Q(k), is approximated as: nl 2 jk (3) Q(k) = A0 + A1 jk + A2 ( jk) +∑A2+n n=1 jk +γ n • Where nl is the number of aerodynamic lags and γn are aerodynamic lag coefficients. 23 Roger’s EOMs • The equations of motion of the complete aeroelastic system then become: $ M −1C M −1K M −1A M −1A ' − − − 3 − nl +2 & I 0 0 0 ) & ) q˙ = & 0 I −Vγ 1 /bI 0 ) q & ) & ) % 0 I 0 −Vγ n /bI ( • Where l 1 1 1 1 M = M − ρb2A , C = C − ρUbA , K = K − ρU 2A , A = − ρU 2A s 2 2 s 2 1 s 2 0 j 2 j • €Usually: n nl = 4, γ n = −1.7kmax 2 , kmax = maximum k of interest € (nl +1) 24 € Roger details • The matrices A0, A1 etc are obtained from equation (3) by performing a least-squares curve fit of Q(k) for several values of k. • If the aeroelastic system has n degrees of freedom, Roger’s equations of motion will have (nl+2)n states. Of those, nln states are aerodynamic states. • There are similar but more efficient schemes for improving the curve fit of Q(k) and reducing nl, e.g. the minimum state method. 25 Practical Aeroelasticity • For an aircraft, the matrix Q(k) is oBtained using a panel method-based aerodynamic model. • The modelling is usually performed by means of commercial packages, such as MSC.Nastran or Z- Aero. • For a chosen set of k values, e.g. k1, k2, …, km, the corresponding Q matrices are returned. • The Q matrices are then used in conjunction with the p-k method to obtain the flutter solution or time-domain responses.
Details
-
File Typepdf
-
Upload Time-
-
Content LanguagesEnglish
-
Upload UserAnonymous/Not logged-in
-
File Pages70 Page
-
File Size-