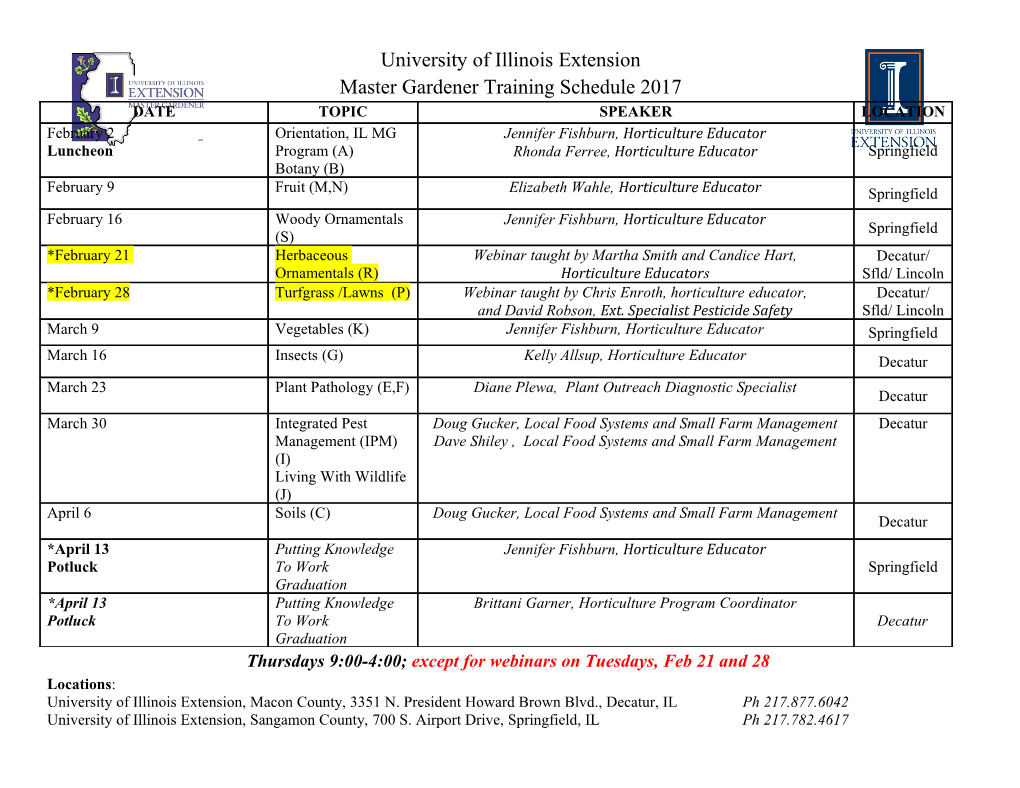
BBA - Bioenergetics 1860 (2019) 433–438 Contents lists available at ScienceDirect BBA - Bioenergetics journal homepage: www.elsevier.com/locate/bbabio Review The mechanism of cyclic electron flow T ⁎ W.J. Nawrockia,b, , B. Bailleula, D. Picotc, P. Cardolb, F. Rappaporta,1, F.-A. Wollmana, P. Joliota a Institut de Biologie Physico-Chimique, UMR 7141 CNRS-UPMC, 13 rue P. et M. Curie, 75005 Paris, France b Laboratoire de Génétique et Physiologie des Microalgues, Institut de Botanique, Université de Liège, 4, Chemin de la Vallée, B-4000 Liège, Belgium c Institut de Biologie Physico-Chimique, UMR 7099 CNRS-UPMC, 13 rue P. et M. Curie, 75005 Paris, France ARTICLE INFO ABSTRACT Keywords: Apart from the canonical light-driven linear electron flow (LEF) from water to CO2, numerous regulatory and Photosynthesis alternative electron transfer pathways exist in chloroplasts. One of them is the cyclic electron flow around Biophysics Photosystem I (CEF), contributing to photoprotection of both Photosystem I and II (PSI, PSII) and supplying extra Plastoquinone ATP to fix atmospheric carbon. Nonetheless, CEF remains an enigma in the field of functional photosynthesis as Cytochrome b f 6 we lack understanding of its pathway. Here, we address the discrepancies between functional and genetic/ Photosystem I biochemical data in the literature and formulate novel hypotheses about the pathway and regulation of CEF Cyclic electron flow based on recent structural and kinetic information. 1. Linear and cyclic electron flow kinetic and methodological standpoint in the light of our report that PGRL1 is not directly transferring electrons from Fd to PQ during CEF Photosynthesis consists of a photoinduced linear electron flow [1]. We further propose a mechanism that can explain how and why the (LEF), where electrons from water are transferred to NADP+. The rate of CEF is not tightly controlled by the redox state of the PQ pool. electron-coupled proton translocation generates a proton motive force across the thylakoid membrane that is used by the CF1-F0 ATP synthase 2. Current view on cyclic electron flow to form ATP. Photoproduced ATP and NAD(P)H then allow CO2 fixation by the Calvin-Benson-Bassham cycle. However, alternative electron CEF was first identified by the Arnon group ([2] and references transfer pathways have been documented in photosynthesis. Most no- therein). These experiments pointed to the existence of cyclic photo- table among them are water-to-water cycles and cyclic electron flow phosphorylation as a pathway of light-dependent ATP phosphorylation, (CEF) around Photosystem I (PSI). All these alternative pathways where the net production or consumption of reducing power was null. translocate extra protons into the lumen without producing reducing Therefore, CEF was defined as a rerouting of reducing equivalents from compounds, thus increasing the ATP/NAD(P)H ratio. The dynamic the acceptor side of PSI back to its donor side. The implication of cyt b6f changes between the rates of LEF and alternative pathways are thus in CEF, also proposed by Arnon and co-workers, was further confirmed critical in conditions where i) NAD(P)H accumulates when the electron by the sensitivity of CEF to Qo site inhibitors [3]. Later attempts to transfer chain is kinetically limited by the NAD(P)H oxidation pathways identify specific CEF transporters - in particular through genetic ap- (carbon fixation and others), and ii) lumen acidification needs to be proaches aimed at the isolation of mutants devoid of CEF - fell short but enhanced to protect the two photosystems via non-photochemical allowed to disclose molecular bases for CEF regulation. These studies led quenching (NPQ) and photosynthetic control at the level of cytochrome to propose two CEF routes, which are considered by most authors to be b6f (cyt. b6f). responsible for the reduction of PQ by PSI acceptors: the NDH-1/NDH- Despite the well-recognized importance of CEF in the regulation of 2-, and PGR5/PGRL1-dependent pathways (e.g. ref. [4]) (Fig. 1). For the photosynthesis, information about its precise mechanism is still lacking. first pathway, the components allowing the transfer of electrons from Not only is our understanding of the regulation of CEF and LEF path- PSI acceptors to the PQ pool are homologous to the respiratory NAD(P) ways scarce, but even the cofactors involved in electron transfer from H:PQ oxidoreductases. In land plant chloroplasts, NDH-1 shares at least Ferredoxin (Fd) to plastoquinone (PQ) remain elusive. Here, we re- 11 subunits with the mitochondrial and bacterial NADH:UQ oxidor- assess current knowledge about CEF pathways, in particular from a eductase (complex I) [5]. Chloroplast NDH-1 complex however lacks an ⁎ Corresponding author at: Department of Physics and Astronomy, Vrije Universiteit Amsterdam, De Boelelaan 1081, 1081HV Amsterdam, the Netherlands. E-mail address: [email protected] (W.J. Nawrocki). 1 Deceased. https://doi.org/10.1016/j.bbabio.2018.12.005 Received 19 August 2018; Received in revised form 8 December 2018; Accepted 8 December 2018 Available online 07 March 2019 0005-2728/ © 2018 Elsevier B.V. All rights reserved. W.J. Nawrocki et al. BBA - Bioenergetics 1860 (2019) 433–438 Fig. 1. A scheme of CEF and chlororespiration, and the molecular actors involved in these processes. PGRL1 (dashed) could almost stoichiometrically bind to cyt. b6f to regulate CEF. Note that chlororespiration rates are so low that the only contribution of this process is observed in darkness [16]. NADH binding module and has been proposed to use Fd as a substrate qE, and thus ΔpH across the thylakoid membrane, leading to their name [6]. It probably pumps additional protons per electron transferred [7], (proton gradient regulation 5 and pgr5-like 1, respectively) [18,19]. similarly to mitochondrial and bacterial complexes I. In some micro- However, despite a plethora of proof of their involvement in the reg- algae, NDH-2 is present instead of NDH-1. It resembles mitochondrial ulation of CEF [4,18,20–24], there is still no clear evidence of their Ndi1 [8], and is a monotopic membrane protein [9] that inserts into the direct role in the transfer of electrons from the PSI acceptors to the PQ stromal leaflet of the thylakoid membrane, and therefore is unable to pool. On the contrary, several observations make this very unlikely. pump protons to the lumen upon oxidation of its substrate, NAD(P)H PGR5 is a small, stroma-soluble protein, binding no cofactors [25], and [10]. Yet, the enzymatic pathway allowing the electron transfer from it is found tethered to the thylakoids by PGRL1. The maximal rate of PSI acceptors back to the electron transfer chain upstream cytochrome CEF does not change in the absence of the former at the beginning of b6f is still a matter of debate. Any legitimate candidate (enzyme or the induction of photosynthesis, showing that while PGR5 is involved in combination of enzymes) for the closing of the cycle must display a fine-tuning of CEF, its presence is not required for a fully efficient Fd:PQ turnover rate in agreement with the highest rates of CEF measured in electron transfer [20]. PGRL1 has been proposed to fulfil the role of the vivo, i.e. ~100 and ~60 electrons per second per PSI in plants [11,12] elusive FQR [26], directly reducing quinones, and could almost stoi- and in green algae (from the companion paper, [1]). chiometrically bind to the fraction of cyt. b6f found in the stroma la- The role of NDH-1 in CEF is doubtful from many perspectives. It is mellae [27]. However, the FQR role is difficult to reconcile with its sub- highly sub-stoichiometric with regards to PSI (1:100 ratio [13]), stoichiometric ratio relative to PSI, with about 0.3 proteins per PSI, or − − − therefore its rate would necessarily need to exceed 104 e ·s 1 per 0.15 PSI 1 if it indeed dimerises in vivo [26]. Crucially, we show in the complex to sustain transitory rates of CEF in plants in the order of a accompanying article [1] that a Chlamydomonas pgrl1 mutant exhibits hundred of electrons per second per PSI [11,12], a value up to 4 orders no differences in the maximal transitory rate of CEF, which would be of magnitude higher than experimentally measured in vivo for this en- expected if PGRL1 was a bona fide CEF Fd:PQ oxidoreductase. Instead, zyme [14]. Experimental data shows that the absence of NDH-1 does we observed that the PSI acceptor side was altered in its contribution to not alter the qE (pH-dependent NPQ) – and therefore its proton- charge recombination within PSI and to the rerouting of reductants pumping contribution is negligible – in plants [15], consistent with its toward CEF, both of which being lower than in the WT. These traits − − − measured rate being in the order of only one tenth (0.1) e ·s 1·PSI 1 in rather argue for an indirect role of PGRL1 in CEF, similarly to that of vivo [14]. Similarly, NDH-2 - the algal counterpart of NDH-1 – runs at PGR5 [28], by regulating either the fate of PSI acceptors or the redox − − − 2.5 e ·s 1·PSI 1 [7,16], a rate which is far too sluggish to sustain the state of the stroma. Such a role would go in line with the observations − − − rate of 60 e ·s 1·PSI 1 for CEF that we report in the companion paper that PGRL1 is required for recruitment of PGR5 into PGRL1/PGR5 [1]. Finally, an NDH-2 mutant only showed limited changes in electron complex [28]. transfer with a slower PQ re-reduction in darkness [10] or in the light, exclusively when the competing PQ-reducing PSII activity was severely 3.
Details
-
File Typepdf
-
Upload Time-
-
Content LanguagesEnglish
-
Upload UserAnonymous/Not logged-in
-
File Pages6 Page
-
File Size-