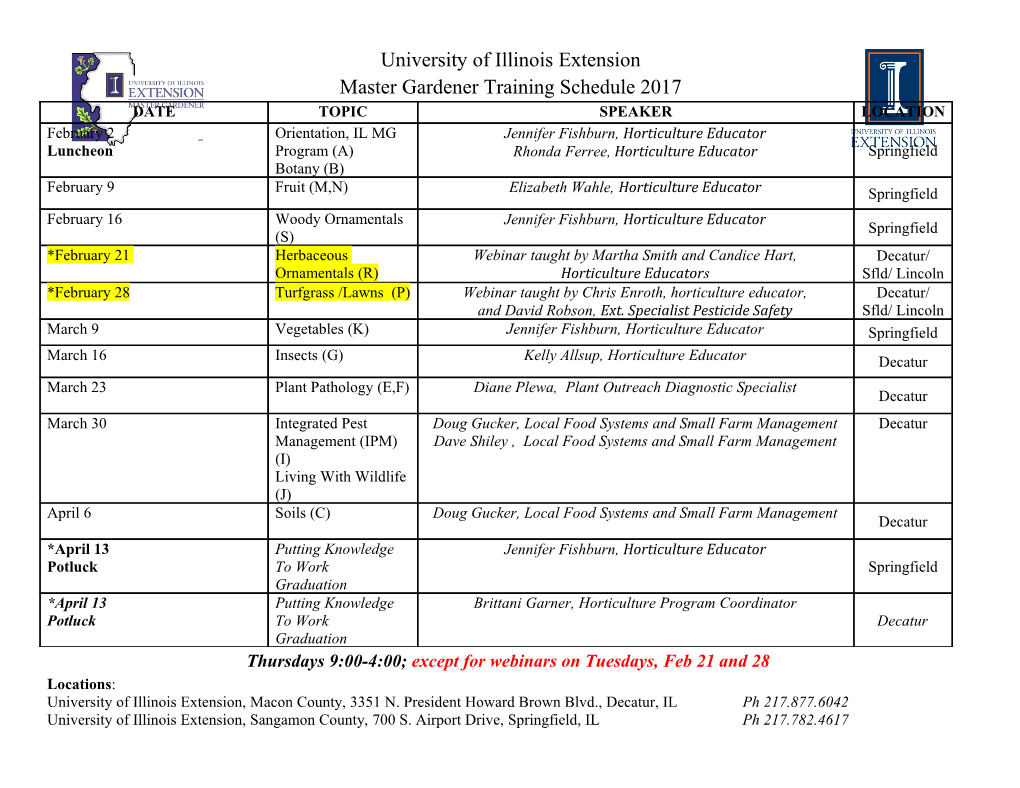
Invasive Species Management and Control: Avian malaria (Plasmodium relictum) 1.0 PREVENTATIVE MEASURES 2.0 GENETIC MEASURES 3.0 CHEMICAL CONTROL 1.0 PREVENTATIVE MEASURES P. relictum presence is linked to mosquito populations, particularly of its main vector Culex quinquefasciatus Say. Thus effective management of avian malaria will “require extensive mosquito control efforts that are guided by the local ecology of the vector Culex quinquefasciatus (Lapointe 2008). To effectively eliminate malaria from a habitat either the vector (mosquito) must be eliminated or prevented from feeding on the bird (USDI and USGS 2005). This is difficult particularly in remote areas in the wet forests of Hawaii where wallows from feral pigs and hollowed out logs of the native apuu ferns provide ample areas of standing water where the mosquito breeds (USDI and USGS 2005). One effective procedure is to reduce the number of potential water catchment containers in order to reduce the mosquito breeding sites available (SPREP Undated). However, in Hawaii attempts to control mosquitoes by larval habitat reduction and larvicide use have been largely unsuccessful. The most common approach used to control mosquito vectors is the application of insecticides (McCarroll and Hemingway 2002 in Liu et al. 2005). However Cx. quinquefasciatus rapidly acquires resistance to many insecticides including organophosphates, carbamates and pyrethroids. Current research is directed towards identifying alternative insecticides, which meet criteria for vector control and show no cross-resistance to pyrethroids (Mosha et al. 2008). Biological control of mosquitoes has become a topic of considerable interest in response to the problem of insecticide resistance. The bacterium Bacillus thuringiensis subsp. israelensis (Bti) is highly effective in controlling larvae of many mosquito species and is the active ingredient for commercial larvicides. Other bacterial larvicides such as B. sphaericus are also used in some countries, and current research is examining the effectiveness of natural predators such as odonate nymphs for mosquito control (e.g. Mandal et al. 2008). More information on management of Culex quinquefasciatus is available on the GISD profile for this species. Vaccination is a potential method of controlling P. relictum. A study by McCutchan et al. (2004) looked at the effects of vaccinating canaries in Baltimore Zoo with a DNA vaccine plasmid encoding the circumsporozoite protein of P. relictum. In the first year after vaccination birds “exhibited a moderate degree of protection against natural infection” with non-vaccinated birds dying in significantly greater numbers than vaccinated birds. In the second year “vaccinated birds were no longer statistically distinguishable for protection against malaria from cages of naïve birds”. Vaccination also had a surprising effect in that it “seemed to interfere with the acquisition of reinfection immunity because in the follow-up year all birds that died were ones that had previously been vaccinated, while those that had acquired immunity as a result of exposure were fully protected”. It seems that the vaccine may have cause a total elimination or significant reduction in parasite load, which interferes with the acquisition of natural immunity. Indeed the introduction of vaccine into areas of avian malaria could actually worsen malaria conditions. This study has particular significance for human malaria (McCutchan et al. 2004) and for high value birds such as African black-footed penguins (Spheniscus demersus). Grim et al. (2004) determined the impact of the same vaccine on penguins in Baltimore Zoo. “Among the vaccinated penguins, the parasitemia rate dropped from approximately 50% to approximately 17% despite intense parasite pressure, as determined by mosquito infection rate. During the year of the vaccine trial, no mortalities due to malaria occurred and no undesirable vaccination side effects occurred” (Grim et al. 2004). 2.0 GENETIC MEASURES Avian malaria is particularly a problem on Hawaii due to its large number of endemic and vulnerable bird species. Current conservation and restoration efforts in Hawaii focus on high elevation areas, where the primary vector Culex quinquefasciatus are absent due to physiological (thermal) limitations (Foster et al. 2007), and thus where the most dense populations of native birds are found (US Fish and Wildlife Service 2003) in Kilpatrick 2006). However recent climate change projections suggest global temperatures will increase by 2°C which could eliminate mosquito-free area (Benning et al. 2002 in Kilpatrick 2006). Thus it is important that management strategies focus on the evolution of resistance to malaria in endemic birds (Kilpatrick 2006). Immunological resistance has been reported for some species on Hawaii. For example elepaio populations on the island of O’ahu persist at low elevations where mosquito and malaria levels are high which indicates resistance, although it has not been clinically demonstrated (Vanderwerf et al. 2006). The Hawaiian amakihi (Hemignathus virens) is also found in dense populations in low-elevation areas in Hawaii (Foster et al. 2007) even though high levels of malaria persist and there is evidence for local transmission (Woodworth et al. 2005). Foster et al. (2007) found that “amakihi in lowland areas are, and have historically been, differentiated from birds at high elevations and had unique alleles retained through time; that is, their genetic signature was not a subset of the genetic variation at higher elevations. We suggest that high disease pressure rapidly selected for resistance to malaria at low elevation, leaving small pockets of resistant birds, and this resistance spread outward from the scattered remnant populations.” Thus the future of amakihi almost certainly lies in the resurgence of its low-elevation populations which contain unique genetic diversity. Foster et al. (2007) conclude that “Determining the conditions that allow for the development of resistance to disease is essential to understanding how species evolve resistance across a landscape of varying disease pressures” and is essential to developing and facilitating resistance to Plasmodium relictum in Hawaiian birds. Some researchers have suggested rodent control to facilitate the evolution of malaria resistance. Rats (Rattus spp.) are a major cause of decline for many Hawaiian birds. Thus it is thought that “rat control may facilitate evolution of disease resistance by providing birds that have greater immunity an increased chance of reproducing, thereby increasing the proportion of resistant birds in each subsequent generation more quickly” (VanderWerf and Smith 2002). The genetic basis of avian malaria resistance in Hawaiian birds is not well understood (Jarvi et al. 2001, 2004), although research suggests that there are several loci involved in susceptibility (Swardson et al. 1997; Burt et al. 2002; Henri et al. 2002 in Kilpatrick 2006). A study by Vanderwerf and Smith (2002) found that controlling rodent populations was extremely effective in increasing reproduction and survival of elepaio on Oahu. Kilpatrick (2006) looked at the effects of controlling rodents on the evolution of resistance to malaria in seven native Hawaiian birds. “Modeling results demonstrated that rodent control at middle, but not high, elevations can facilitate the evolution of resistance to malaria in several species of Hawaiian birds”. Although it seems unlikely that resistance is governed by a single recessive allele if current genetic work (Jarvi et al. 2001, 2004) determines that this is the case for some species, then a different strategy would be more effective as resistance would be unlikely to be maintained in a population. Strategies may involve breeding captive populations of resistant individuals and introducing them to areas where they would be unlikely to breed with susceptible individuals, as this would create susceptible heterozygotes. Kilpatrick points out that the models presented ignore the evolution of P. relictum. It is well known that pathogens can evolve at much higher rates than their hosts, and thus “evolution for increased virulence by the parasite could counteract evolution for resistance in the host population” (Kilpatrick 2006). Avian malaria (Plasmodium relictum) can also have some unexpected impacts on avian populations. Acute infections could act as a selective agent by removing less fit individuals from a population. A study on Amakihi in Hawaii found that “chronic malaria infections in male and female parents did not significantly reduce reproductive success. Indeed pairs with infected males had higher nesting success rate than those with uninfected males, and offspring that had at least one parent that survived the acute phase of malaria infection had a greater chance of being sighted the following year (although this was based on small sample sizes and may be due to lower dispersal of infected birds). Overall the “reproduction and survival of infected birds were sufficient for a per-capita population growth >1, which suggests that chronically infected Hawaii Amakihi could support a growing population” (Kilpatrick et al. 2006). CHEMICAL CONTROL: Captive bird populations may be treated with pharmaceuticals such as chloroquine and primaquine to cure the disease (Cranfield et al. Undated). .
Details
-
File Typepdf
-
Upload Time-
-
Content LanguagesEnglish
-
Upload UserAnonymous/Not logged-in
-
File Pages4 Page
-
File Size-