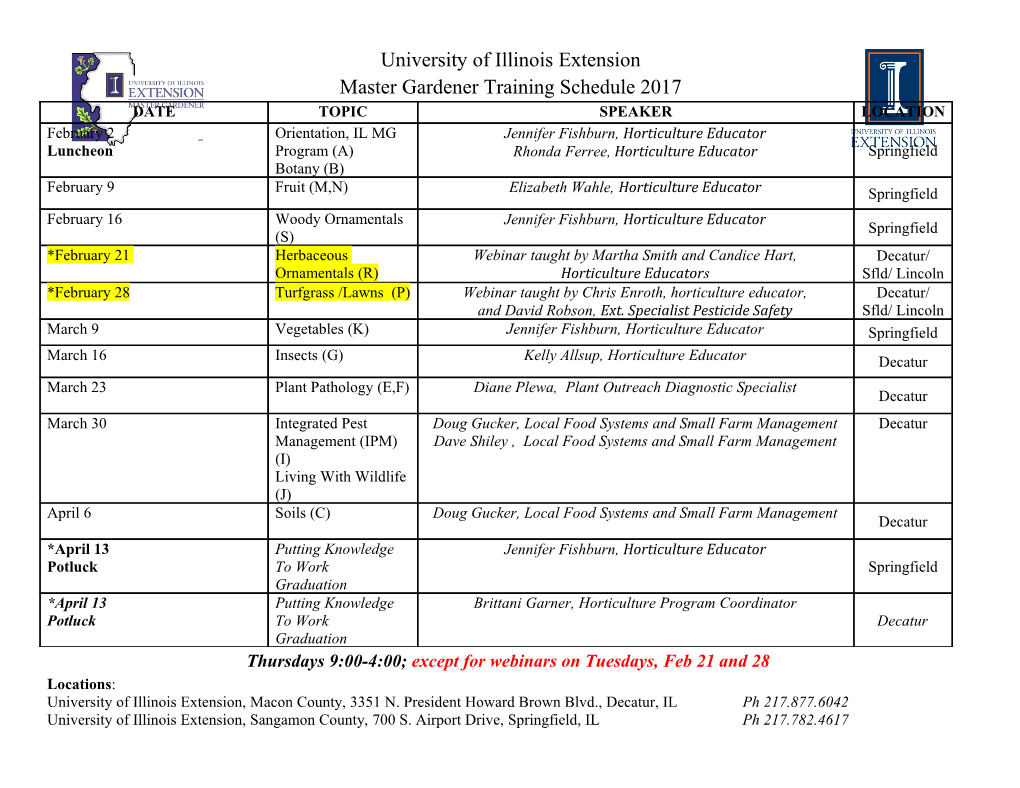
Tectonophysics, 140 (1987) 49-63 49 Elsevier Science Publishers B.V., Amsterdam - Printed in The Netherlands The crustal structure of the axis of the Great Valley, California, from seismic refraction measurements W. STEVEN HOLBROOK ’ and WALTER D. MOONEY * ’ Geophysics Department, Stanford University, Stanford, CA 94305 (U.S.A.) ’ U.S. Geological Survey, MS 977, 345 Middlefield Road, Menlo Park, CA 94025 (U.S.A.) (Received March 18,1986; accepted June 151986) Abstract Holbrook, W.S. and Mooney, W.D., 1987. The crustal structure of the axis of the Great Valley, California, from seismic refraction measurements. In: S. Asano and W.D. Mooney (Editors), Seismic Studies of the Continental Lithosphere. Tectonophysics, 140: 49-63. In 1982 the U.S. Geological Survey collected six seismic refraction profiles in the Great Valley of California: three axial profiles with a maximum shot-to-receiver offset of 160 km, and three shorter profiles perpendicular to the valley axis. This paper presents the results of two-dimensional raytracing and synthetic seismogram modeling of the central axial profile. The crust of the central Great Valley is laterally heterogeneous along its axis, but generally consists of a sedimentary section overlying distinct upper, middle, and lower crustal units. The sedimentary rocks are 3-5 km thick along the profile, with velocities increasing with depth from 1.6 to 4.0 km/s. The basement (upper crust) consists of four units: (1) a 1.0-1.5 km thick layer of velocity 5.4-5.8 km/s, (2) a 3-4 km thick layer of velocity 6.0-6.3 km/s, (3) a 1.5-3.0 km thick layer of velocity 6.5-6.6 km/s, and (4) a laterally discontinuous, 1.5 km thick layer of velocity 6.8-7.0 km/s. The mid-crust lies at 11-14 km depth, is 5-8 km thick, and has a velocity of 6.6-6.7 km/s. On the northwest side of our profile the mid-crust is a low-velocity zone beneath the 6.8-7.0 km/s lid. The lower crust lies at 16-19 km depth, is 7-13 km thick, and has a velocity of 6.9-7.2 km/s. Crustal thickness increases from 26 to 29 km from NW to SE in the model. Although an unequivocal determination of crustal composition is not possible from P-wave velocities alone, our model has several geological and tectonic implications. We interpret the upper 7 km of basement on the northwest side of the profile as an ophiolitic fragment, since its thickness and velocity structure are consistent with that of oceanic crust. This fragment, which is not present lo-15 km to the west of the refraction profile, is probably at least partially responsible for the Great Valley gravity and magnetic anomalies, whose peaks lie about 10 km east of our profile. The middle and lower crust are probably gabbroic and the product of magmatic or tectonic underplating, or both. The crustal structure of the Great Valley is dissimilar to that of the adjacent Diablo Range, suggesting the existence of a fault or suture zone throughout the crust between these provinces. Introduction quence and overlying Cenozoic units, form an asymmetric syncline with a steeply-dipping west The Great Valley of California is a 700~km-long limb and a gently-dipping east limb (e.g., Dickin- by 100~km-wide sedimentary basin situated be- son, 1981). The Great Valley is of geologic interest tween the granitic and metamorphic terrane of the not only as a prolific oil-bearing province, but also Sierra Nevada and the Franciscan subduction as a key to the tectonic evolution of central Cali- complex of the Coast Ranges. The sedimentary fornia. rocks of the Great Valley, which include the late Recent geological work, most notably in the Jurassic to late Cretaceous age Great Valley Se- Coast Ranges (e.g., Blake et al., 1984) and Sierra 0040-1951/87/$03.50 0 1987 Elsevier Science Publishers B.V. 50 Nevada (e.g., Day et al., 1985), has greatly im- margin; still, many important questions remain proved our understanding of the Mesozoic and unanswered. The outstanding problems include: Cenozoic evolution of the California continental (1) the composition of the basement beneath the / / / Reflection ProfileD / I Shotpolnt 14 *** _.*- Arts Of YaSnOttC *.** Anomclly *.** Fig. 1. Location map of Great Valley, California, showing refraction lines, reflection lines, generalized geology, and the approximate location of the peak of the Great Valley magnetic anomaly (Cady, 1975). Qt = Quaternary sediments; Terr = Tertiary sediments; TV = Tertiary volcanics; arm = ultramafics; K = Cretaceous sedimentary rocks, largely the Great Valley Sequence: Frnn = Jurassic and Cretaceous Franciscan melange; MGr = Mesozoic granites and granodiorites of the Sierra Nevada: h4.r = undifferentiated Mesozoic rocks, including Sierra greenstone belt. SAF-San Andreas Fault; St--Stockton; MO-Modesto; Me-Merced; Fr-Fresno. 51 Great Valley, (2) the existence and location of the such as the Great Valley. Figure 3 shows a gen- postulated contact between continental Sierra eralized travel-time curve for the major phases Nevada crust and oceanic-affinity crust further observed on the three shots. Not all of these west, (3) the cause of the Great Valley magnetic general phases are present on all profiles, however, and gravity anomalies (Cady, 1975; Griscom, 1982; indicating significant lateral variation along the Griscom and Jachens, 1986), (4) the relationship axis of the valley. The following section describes between the Coast Range Ophiolite and the the phases observed on the three shots. Smartville Ophiolite of the western Sierra Nevada, and (5) the crustal thickness and composition of Shotpoint I4 the deep crust of the Great Valley. Because the answers to these questions are concealed beneath The maximum shot-receiver offset of SP14 is the sediments of the Great Valley, geophysical long enough (160 km) to record refracted and methods must be used to investigate them. reflected arrivals from the entire crust and upper- In order to pursue these questions, the U.S. most mantle. At near offset, first arrivals with Geological Survey (USGS) conducted a seismic apparent velocities of about 1.8 to 4.0 km/s (A refraction survey in June, 1982, in the Great Val- and B, Fig. 4) represent energy refracted in the ley between the latitudes of Stockton and Fresno sedimentary section. Behind these arrivals a prom- (Fig. 1). The refraction survey, part of a larger inent basement reflection (C, Fig. 4) asymptotes seismic refraction and reflection program in the to an apparent velocity of 4.0 km/s, which is Great Valley, consisted of three axial lines, with a inferred as the velocity at the base of the sedimen- maximum shot-to-receiver offset of 165 km, and tary section. Control on the velocity gradients three shorter cross-lines perpendicular to the val- within the sedimentary section comes from promi- ley axis. This paper presents the results of an nent multiple refractions (AA, Fig. 4) which arrive interpretation of the central axial refraction pro- at twice the travel time and distance of first arrivals file. We begin with a description of the data, from the sediments and basement. Beyond 15 km, present a model consistent with the observed first arrivals refracted from three distinct upper seismic phases, then discuss some of the geological crustal layers have apparent velocities of 6.3, 6.6, implications of that model. and 7.2 km/s (branches E, F, and G, Fig. 4); however, reversals from the other shotpoints indi- Refraction data cate that these are all updip, i.e. higher-than-ac- tual, velocities. At least two strong upper-crustal The Great Valley central axial profile consists reflections arrive behind these upper-crustal re- of three shotpoints (SP14, 15, and 16) along a 180 fractions. km-long line (Fig. 1). Seismic energy was gen- Arrivals from the deep crust and upper mantle erated by 800- to 1600-kg chemical explosions and yield apparent velocities of 7.0 (I, Fig. 4) and 8.1 recorded on FM analog tape by 120 portable km/s (J, Fig. 4) respectively, for these layers. seismographs (Healy et al., 1982). Final record These velocities are unreversed, since the maxi- sections were filtered l-10 Hz and plotted in mum offset on SP16 is too short to record these normalized (Fig. 2) and true amplitude format. phases (cf. Fig. 2). The Moho reflection (1, P,P) is The refraction data provide information on the prominent beyond 75 km and is the highest-ampli- compressional-wave velocity and structure of the tude phase on true-amplitude records. Beyond 110 entire crust and uppermost mantle. The shot spac- km, P,P is immediately followed by high-ampli- ings of 30 km and 50 km provide good reversed tude, ringing arrivals which may be peg-leg multi- control on the sedimentary and upper crustal ples from within the crust or reflections from velocities, but the velocities of the lower crust and within the mantle, which we have not modeled. A upper mantle are unreversed. The observed phases delay of about 1.0 set of P,,,P (I) with respect to are generally similar on the three shots, as would the extrapolation of the 7.2 km/s upper-crustal be expected on an axial line in a linear province phase (G), combined with the rapid amplitude 52 Normalized Amplitude SE 6 5 ri 3 2 1 0 -1 -2 UO 60 80 -UO -20 D 20 '40 60 80 100 120 Distance (km) 6 6 5 5 3 rl 4 w: : 1c3 2 ;; A 1 - 1 0 0 -1 -i -2 L-2 0 20 40 60 80 100 t20 160 Dirtance (km) Fig.
Details
-
File Typepdf
-
Upload Time-
-
Content LanguagesEnglish
-
Upload UserAnonymous/Not logged-in
-
File Pages15 Page
-
File Size-