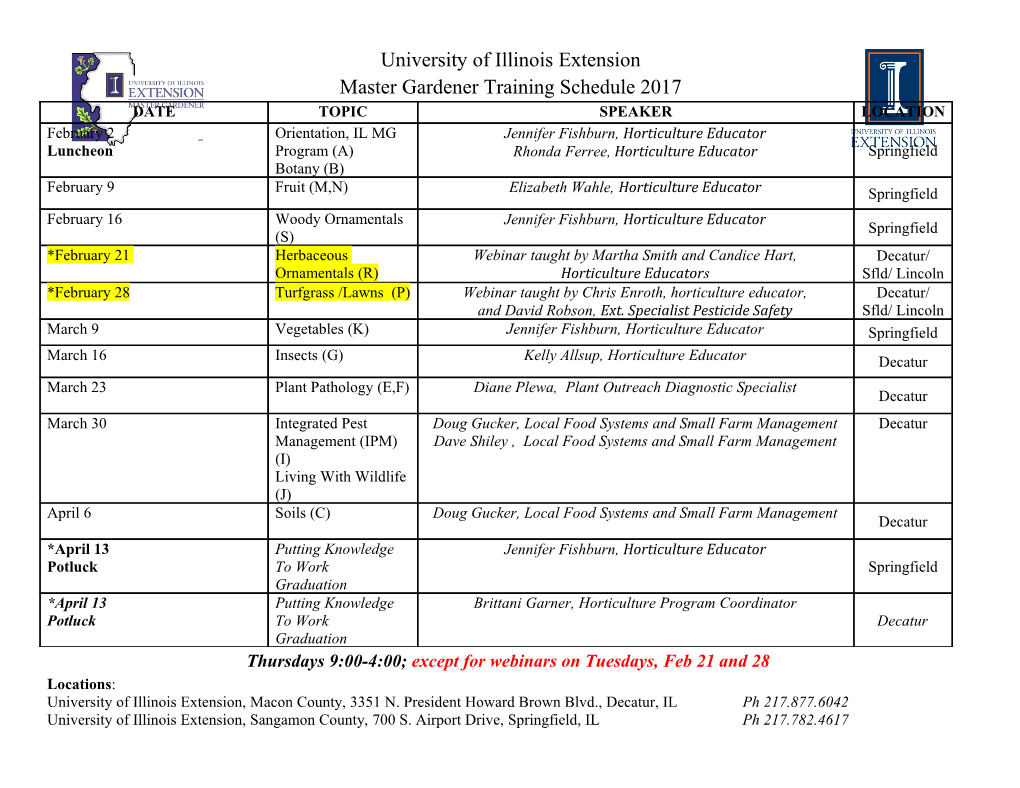
Available online at www.sciencedirect.com Journal of the European Ceramic Society 29 (2009) 2185–2191 Characterisation of fluorine containing glasses and glass-ceramics by 19F magic angle spinning nuclear magnetic resonance spectroscopy R.G. Hill a, R.V. Law b, M.D. O’Donnell a,b,∗,J.Hawesb, N.L. Bubb c, D.J. Wood c, C.A. Miller d, M. Mirsaneh e, I. Reaney e a Department of Materials, Imperial College, London SW7 2BP, UK b Department of Chemistry, Imperial College, London SW7 2BP, UK c Leeds Dental Institute, University of Leeds, Leeds LS2 9LU, UK d The School of Clinical Dentistry, The University of Sheffield, Sheffield S10 2TA, UK e Department of Engineering Materials, The University of Sheffield, Sheffield S1 3JD, UK Received 10 July 2008; received in revised form 18 December 2008; accepted 13 January 2009 Available online 28 February 2009 Abstract 19F magic angle spinning nuclear magnetic resonance (MAS-NMR) spectroscopy was used to characterise the local environment of fluorine in three types of fluorine containing glass-ceramics. The glass-ceramic compositions studied included four that crystallised to fluorcanasite, one which crystallised to barium fluorphlogopite and one which crystallised to fluorrichterite. In the fluorcanasite glasses, prior to crystallisation, the fluorine was present solely as an F–Ca(n) species whilst following crystallisation it was also present as an F–Ca(n) species in the fluorcanasite phase and in those glasses containing AlPO4 it was also present as an F–Ca(n) species in fluorapatite. In the fluorrichterite and fluorphlogopite glasses the fluorine was present predominantly as F–Mg(3) and following crystallisation it was also present as F–Mg(3) in the fluorrichterite and fluorphlogopite phases. In all these glass-ceramics fluorine appears to be preferentially associated with the cations of highest charge to size ratio and the local environment of fluorine in the glass and the crystal phase is almost identical. © 2009 Published by Elsevier Ltd. Keywords: Glass-ceramic; NMR; Oxyfluoride 1. Introduction next nearest neighbours, bond lengths and bond angles. Whilst nuclei such as 29Si, 27Al, 7Li and 31P have been widely used to There are a significant number of synthetic glass-ceramics characterise minerals, glasses and glass-ceramics,10,11 there are that have been developed based on fluorine containing crys- very few studies of 19F magic angle spinning nuclear magnetic 1–3 tal phases. Examples include fluorapatite (Ca5(PO4)3F), resonance (MAS-NMR) applied to glasses and glass-ceramics. 4–6 potassium fluorphlogopite (K2Mg6Si6Al2O18F4), flu- Development of MAS probes with spinning rates > 20 kHz have 5,7,8 orrichterite (KNaMg5Si8O22F2) and fluorcanasite contributed to reduction in line broadening and minimising the 9 12 19 ((Na,K)6Ca5Si12O30F4). These phases are the fluorine need for multiple pulse techniques. The F nucleus is 100% analogues of the natural hydroxyl containing mineral phases. abundant and is the most sensitive nucleus after hydrogen, with The fluorine analogues are utilised for two reasons; fluorine has a spin = 1/2 and with a large degree of chemical shift anisotropy. a similar charge to size ratio as a hydroxyl ion and fluorine is The chemical shift of 19F ranges from +500 ppm to −500 ppm easier to retain in the glass melt. thus the chemical shift of fluorine is very sensitive to its chem- Solid state MAS-NMR is a powerful technique for charac- ical environment. The observed 19F chemical shift has been terising solids and gives information on co-ordination states, shown to correlate with the fluorine-cation distance in alkali metal fluorides.13 − ∗ Different aspects affecting the co-ordination of F in Corresponding author. Current address: Bioceramic Therapeutics Ltd., Lon- F-bearing silicates and aluminosilicates have also been don, UK. 13–17 E-mail address: [email protected] (M.D. O’Donnell). examined. An investigation into the competition between 0955-2219/$ – see front matter © 2009 Published by Elsevier Ltd. doi:10.1016/j.jeurceramsoc.2009.01.009 2186 R.G. Hill et al. / Journal of the European Ceramic Society 29 (2009) 2185–2191 Table 1 Composition of the glasses studied in mole%. Na2OK2O BaO CaO CaF2 MgO MgF2 Al2O3 P2O5 SiO2 Can 1 10.00 5.00 0.00 15.00 10.00 0.00 0.00 0.00 0.00 60.00 Can 2 9.76 4.88 0.00 14.63 9.76 0.00 0.00 1.22 1.22 58.54 Can 3 9.52 4.76 0.00 14.29 9.52 0.00 0.00 2.38 2.38 57.14 Can 4 3.80 5.10 0.00 19.20 10.30 0.00 0.00 0.00 0.00 61.60 BFP 0.00 0.00 6.52 2.61 0.00 27.25 13.04 7.68 0.87 42.03 F1 3.33 3.33 0.00 0.00 6.62 33.35 0.00 0.00 0.00 53.37 cations of different field strengths to form F–M(n) species12 (FAP) glass-ceramics and mixed calcium/strontium fluorapatite demonstrates that F preferentially bonds to higher field-strength glass-ceramics.20 modifier cations. In another study, NMR analysis was performed on alumino-silicate-lanthanum fluoride glasses. As well as LaF3 2. Experimental species forming there was also evidence of Si–F and Al–F bond- ing. Zeng and Stebbins17 have investigated the reduction of 2.1. Binary fluoride salts bridging oxygens (BOs) and formation of Al–F in aluminosili- − cates when F is added and attempted to establish how this is Reagent grade CaF2, SrF2, MgF2 and BaF2 were obtained dependent on the chemical composition of the glass. The pro- and used to construct a reference plot of chemical shift against portion of non-bridging oxygens (NBOs) upon addition of F−, the fluoride ion–metal cation distance. in conjunction with the amount and type of modifying cations and competition between modifying cations, Al and Si for F− 2.2. Glass compositions ions, all disrupt the silicate and aluminosilicate network. This in turn affects the macroscopic properties of the glass. It is still Glasses were melted with reagent grade SiO2,Na2CO3, not understood which of these factors is dominant in deter- K2CO3, CaCO3, CaF2,P2O5,Al2O3, BaCO3, MgO and MgF2. mining how F− co-ordinates and this aspect requires further The nominal glass compositions are given in Table 1 and further investigation. details of processing can be found in the references below. They A major drawback to 19F MAS-NMR is that parts of the consist of: NMR instrument, probe and rotor are often constructed from • machinable ceramic. For example a fluormica glass-ceramic in Four canasite glass-ceramics (Can 1–4), one based on the sto- the probe or the fluorinated polymer rotor cap. There is thus ichiometry of fluorcanasite (Na4K2Ca5Si12O30F4), another a considerable 19F background signal. However with care this two with 0.25 and 0.5 M additions of Al2O3 and P2O5 and 21–23 signal can be eliminated by a number of background techniques. finally a calcium oxide rich fluorcanasite. • The MAS speed of the rotor can also be a problem, due to overlap A fluorphlogopite glass-ceramic (BFP) based on the of spinning sidebands with the central resonance in the material ternary composition 90% barium fluorphlogopite making quantitative characterisation of species difficult. This is (Ba0.5Mg3Si3AlO10F2), 4% cordierite (Mg2Al4Si5O18), 6% 24 further complicated by dipolar broadening in crystalline materi- tricalcium phosphate (Ca3(PO4)2). • als and exacerbated by the peak broadening seen in amorphous A stoichiometric fluorrichterite (KNaMg5Si8O22F2) glass- 25 materials. This paper characterises three types of glass-ceramic ceramic composition termed F1. systems based on; fluorphlogopite (FP), fluorrichterite (FR), and fluorcanasite (FC) using 19F MAS-NMR. Previous studies 2.3. Heat treatments by Stamboulis et al.18,19 have investigated calcium fluorapatite The heat treatments for the glass-ceramics are summarised in Table 2. Table 2 2.4. 19F MAS-NMR Heat treatments used for the glass-ceramics. ◦ −1 Glass-ceramic Heating rate ( C min ) Heat treatment The 19F measurements were conducted at a resonance Can 1 10 695 ◦C 1 h and 780 ◦C1h frequency of 188.29 MHz, using an FT-NMR spectrometer Can 2 10 720 ◦C 1 h and 825 ◦C1h (DSX-200, Bruker, Germany). The spinning rate of the sam- ◦ ◦ Can 3 10 750 C 1 h and 850 C1h ple at the magic angle was 15 kHz which would position the Can 4a 5 650 ◦C2h ◦ spinning sidebands around ±80 ppm from the central reso- Can 4b 5 850 C2h 19 19 BFP 10 644 ◦C 2 h and 1225 ◦C5h nance. The recycle time was 120 s for F. The F spectra were F1a 5 650 ◦C4h recorded using DEPTH pulse sequence to suppress the probe F1b 5 700 ◦C4h background.26 The reference material for the chemical shift (in F1c 5 800 ◦C4h ppm) was CaF2 taken as −108 ppm relative to the more common F1d 5 900 ◦C4h standard of CFCl3. R.G. Hill et al. / Journal of the European Ceramic Society 29 (2009) 2185–2191 2187 Table 3 19F chemical shifts of crystalline species. Phase δ (ppm) Refs. Fluorapatite −103 [18–20,33] Fluorite −108 [27] Fluorcanasite −116 This work NaF −225 [27] Si–F −130 [12] Al–F–Ca(n) −150 [17] MgF2 −170 [27] Al–F–Mg(n) −150 [35] Fluorphlogopite −178 [36] Fluorrichterite −174 This work 18–20,33 Fig. 1. Chemical shift against F-cation distance open diamonds values taken rapatite, strontium fluorapatite from previous studies are from the literature. FP = fluorphlogopite and FAP = fluorapatite. ♦ = data from27: shown on the plot in Fig. 1 and Table 3 and were used to generate Zn = ZnF2, Mg=MgF2, Cd = CdF2, Ca = CaF2, Sr = SrF2 and Pb = PbF2 and the line of best fit in addition to the NMR data on binary fluoride Ba = BaF2.
Details
-
File Typepdf
-
Upload Time-
-
Content LanguagesEnglish
-
Upload UserAnonymous/Not logged-in
-
File Pages7 Page
-
File Size-