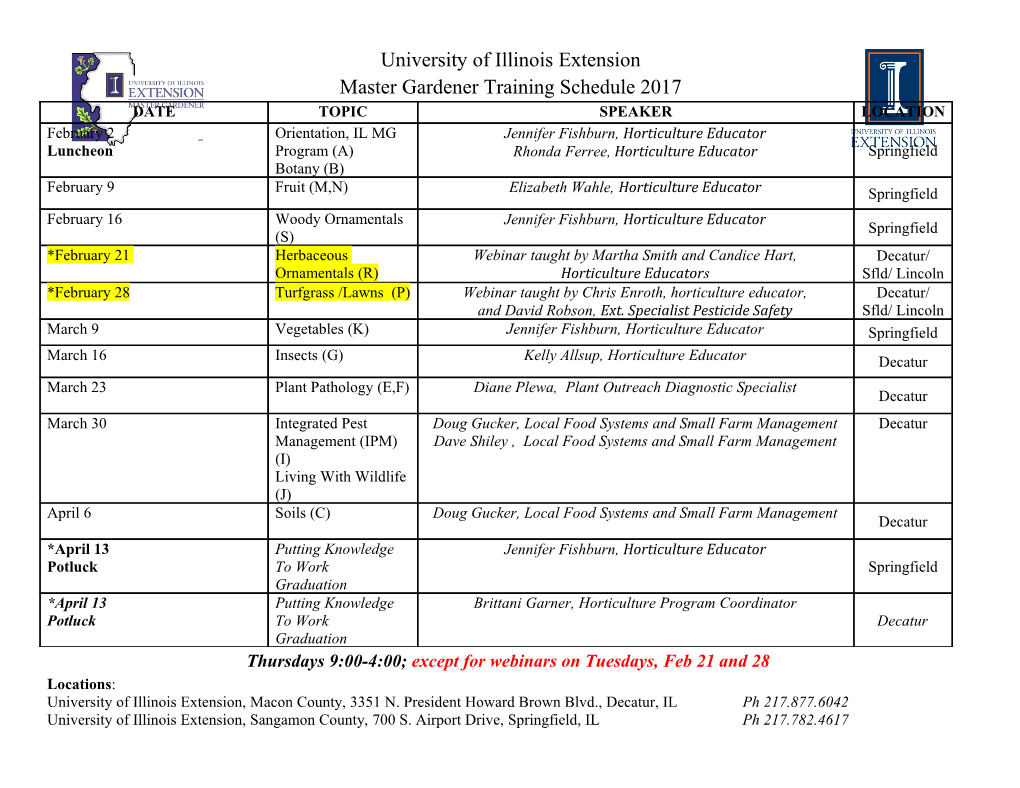
1. MiniBooNE 2. Beam Observation of a Significant Excess of Electron-Like Events3. Detector 4. Oscillation in the MiniBooNE Short-Baseline Neutrino Experiment5. Discussion arXiv:1805.12028 outline 1. MiniBooNE neutrino experiment 2. Booster Neutrino Beamline (BNB) 3. MiniBooNE Detector 4. Oscillation canDiDate search 5. Discussion Teppei Katori for the MiniBooNE collaboration Queen Mary University of London HEP seminar, UCL, UK, June 14, 2018 13/06/18 1 1. MiniBooNE 2. Beam 3. Detector 4. Oscillation 5. Discussion 1. MiniBooNE neutrino experiment 2. Booster Neutrino Beamline (BNB) 3. MiniBooNE Detector 4. Oscillation canDidate search 5. Discussion 13/06/18 2 1. MiniBooNE 2. Beam 3. Detector 4. Oscillation 5. Discussion 13/06/18 3 PHYSICAL REVIEW LETTERS 120, 141802 (2018) signature of dark matter annihilation in the Sun [5,6]. 1. MiniBooNE Despite the importance of the KDAR neutrino, it has never MiniBooNE 2. Beam been isolated and identified. 3. Detector 86 m 4. Oscillation In the charged current (CC) interaction of a 236 MeV νμ background KDAR 12 − NuMI 5. Discussion (νμ C → μ X), the muon kinetic energy (Tμ) and closely beam decay pipe related neutrino-nucleus energy transfer (ω E − E ) horns ν μ target distributions are of particular interest for benchmarking¼ absorber neutrino interaction models and generators, which report widely varying predictions for kinematics at these tran- 5 m 675 m sition-region energies [7–14]. Traditionally, experiments 40 m are only sensitive, at best, to total visible hadronic energy FIG. 1. The NuMI beamline and various sources of neutrinos since invisible neutrons and model-dependent nucleon that reach MiniBooNE (dashed lines). The signal KDAR neu- removal energy corrections prevent the complete trinos (solid line) originate mainly from the absorber. reconstruction of energy transfer [16]. The measurements reported here, therefore, provide a unique look at muon kinematics and the relationship to neutrino energy in the antineutrino modes, since KDAR production from the few hundreds of MeV range, highly relevant for both absorber is not dependent on the polarization of the horns. elucidating the neutrino-nucleus interaction and performing However, the background νμ and ν¯μ event rate is predicted low energy precision oscillation measurements at short to be about 30% lower in the antineutrino mode. We use data taken in this configuration from 2009 2011, corre- [17–19] and long baselines [20]. – sponding to 2.62 × 1020 protons on the NuMI target. The MiniBooNE detector uses 445 tons (fiducial volume) PRL120(2018)141802 of mineral oil and 1280 photomultiplier tubes (PMTs), with The focus of this analysis is on reconstructing KDAR- an additional 240 PMTs instrumenting a veto region, to like low energy νμ CC events. A simple detector observ- identify neutrino events originating from the Booster able, PMThits5ns, defined as the number of PMT hits Neutrino Beamline (BNB) and Neutrinos at the Main multiplied by the fraction of light detected in the first 5 ns Injector (NuMI) neutrino sources. The experiment has after correcting for vertex position, is used to reconstruct Tμ reported numerous oscillation and cross section measure- in selected events featuring (1) an electron from muon ments and new physics searches since data taking began in decay, noting that about 7.8% of μ− capture on nuclei [26], 2002 [17]. For this analysis, we consider the charge and time (2) a lack of veto activity, and (3) a reconstructed distance data of PMT hits collected during the NuMI beam spill. NuMI between the end point of the primary track and the muon provides an intense source of KDAR neutrinos at MiniBooNE decay vertex of < 150 cm. This detector observable is in a somewhat indirect way. The 96 cm, 2.0 interaction length meant to isolate the muon via its characteristic prompt NuMI target allows about 1=6 of the primary NuMI protons Čerenkov light, as compared to the delayed scintillation- (120 GeV) to pass through to the beam absorber [21], 725 m only light (τ 18 ns) from the below-threshold hadronic ¼ downstream of the target and 86 m from the center of part of the interaction. According to the NUWRO neutrino MiniBooNE. The aluminum-core absorber, surrounded by event generator [12], only 14% of muons created in concrete and steel, is nominally meant to stop the remnant 236 MeV νμ CC events are expected to be produced with hadrons, electrons, muons, and gammas that reach the end of MiniBooNEenergy less than keep 39 MeV, providing the Čerenkov threshold for 13/06/18 4 the decay pipe. Interactions of primary protons with the highmuons impact in MiniBooNE results! mineral oil. KDAR-induced muons PRL118(2017)221803 absorber provide about 84% of the total KDAR neutrinos are expected to populate a “signal region,” defined as from NuMI that reach MiniBooNE. Predictions from FLUKA 0–120 PMThits5ns and representing Tμ in the range [22,23], MARS [24],andGEANT4 [25] for kaon production at 0–115 MeV. Because of the kinematics of 236 MeV νμ the absorber vary significantly, from 0.06–0.12 KDAR CC events, no signal is expected elsewhere, which is con- νμ/proton on target. The background to the KDAR signal, sidered the “background-only region” (>120 PMThits5ns). νμ and ν¯μ CC events which produce a muon in the 0–115 MeV Although the signal muon energy range considered for this range, originates mainly from pion and kaon decay in flight measurement is lower than past MiniBooNE cross section near the target station and in the upstream-most decay pipe. analyses featuring νμ=ν¯μ [27–33], the energy and timing The non-KDAR νμ and ν¯μ flux from the absorber, dominated distributions of MiniBooNE’s vast calibration sample of by decay-in-flight kaons (Kμ3 and Kμ2) with a comparatively 0–53 MeV electrons from muon decay provide a strong small charged pion component, is expected to contribute at benchmark for understanding the detector’s response to the few-percent level based on a GEANT4 simulation of the low energy muons in terms of both scintillation and beamline. Figure 1 shows a schematic of the NuMI beamline Čerenkov light. Further, a scintillator “calibration cube” in and its relationship to MiniBooNE. the MiniBooNE volume at a 31 cm depth, used to form a very The KDAR event rate at MiniBooNE is expected to be pure sample of tagged 95 4 MeV cosmic ray muons, similar in both NuMI’s low-energy neutrino and shows excellent agreement betweenÆ data and Monte Carlo 141802-2 LSND, PRD64(2002)112007 1. MiniBooNE � 2. Beam � � ⟶ � = ���+2����+ 1.27Δ�+ 3. Detector 1. LSND experiment $ & 4. Oscillation � 5. Discussion LSND experiment at Los Alamos observed excess of anti-electron neutrino events in the anti-muon oscillation + n µ ¾¾¾¾®n e + p ® e + n neutrino beam. n + p ® d + g 87.9 ± 22.4 ± 6.0 (3.8.s) L/E~30m/30MeV~1 LSND signal + + p ®n µ + µ + + µ ® e +n e +n µ 13/06/18 5 LSND, PRD64(2002)112007 1. MiniBooNE 2. Beam 3. Detector 1. LSND experiment 4. Oscillation 5. Discussion 3 types of neutrino oscillations are found: LSND neutrino oscillation: Dm2~1eV2 Atmospheric neutrino oscillation: Dm2~10-3eV2 Solar neutrino oscillation : Dm2~10-5eV2 But we cannot have so many Dm2! 2 2 2 Dm13 ≠ Dm12 + Dm23 ns nt nµ ne LSND signal indicates 4th generation neutrino, but we know there is no additional flavor from Z-boson decay, so it must be sterile neutrino MiniBooNE is designed to have same L/E~500m/500MeV~1 to test LSND Dm2~1eV2 16/07/18 6 1. MiniBooNE � 2. Beam � � ⟶ � = ���+2����+ 1.27Δ�+ 3. Detector 1. MiniBooNE experiment $ & 4. Oscillation � 5. Discussion Keep L/E same with LSND, while changing systematics, energy & event signature; MiniBooNE is looking for the single isolated electron like events, which is the signature of ne events oscillation − νµ → νe + n → p + e oscillation p n e+ ν µ → νe + → + FNAL Booster target and horn decay region absorber dirt detector € K+ p+ ??? Booster nµ ® ne primary beam secondary beam tertiary beam (protons) (mesons) (neutrinos) MiniBooNE has; - higher energy (~500 MeV) than LSND (~30 MeV) - longer baseline (~500 m) than LSND (~30 m) 13/06/18 7 1. MiniBooNE 2. Beam 3. Detector 1. MiniBooNE is extremely influential! – Tools 4. Oscillation 5. Discussion MiniBooNE: NIMA608(2009)206 fitQun: Flux systematic error: MiniBooNE: PRD79(2009)072002 Likelihood-based Cherenkov ring - Errors are derived directly from hadron production fitter, the main reconstruction data (spline fit), not any flux model. used by Super-Kamiokande - Event weighted with multiverse simulation to make (LSNDàMiniBooNEàSuperK). a smooth covariance matrix with taking account all correlations correctly. Sanford-Wang fit error data error Online remote shift: - <1 event per minute - Even ACNET became web interface after this! - Almost all neutrino experiments at Fermilab adapted online remote shift, including NOvA, MicroBooNE, MINERvA, etc 13/06/18 8 1. MiniBooNE 2. Beam 3. Detector 1. MiniBooNE is extremely influential! – Offsprings 4. Oscillation 5. Discussion Argonne: Zelimir MSU: Kendall Djurcic (PD) Mahn (PhD) Lancaster: Jarek Nowak (PD) Fermilab: Chicago: Dave Yale: Bonnie Jen Raaf (PhD) Schmitz (PhD) Fleming (PD) Fernanda Garcia (PD) Zarko Pavlovic (PD) Royal Holloway: Chris Polly (PD) Jocelyn Monroe (PhD) Sam Zeller (PD) Columbia: Georgia Karagiorgi (PhD) Queen Mary: Teppei Katori (PhD) SLAC: Hiro Tanaka (PD) IHEP: Jun Stony Brook: Mike Cao (PD) Wilking (PhD) Imperial: Morgan Caltech: Ryan Wascko (PD) Patterson (PhD) Pittsburgh: Brian Batell (PD) IFIC: Michel NMSU: Robert Sorel (PhD) Cooper (PD) Virginia Tech: Jon Link (PD) Colorado: Eric Zimmerman (PD) LSU: Martin Florida: Heather Tzanov (PD) Ray (PD) UNAM: Alexis Aguilar Arevalo (PhD) …and more are coming! 13/06/18 9 MiniBooNE: PRD81(2010)092005 1.
Details
-
File Typepdf
-
Upload Time-
-
Content LanguagesEnglish
-
Upload UserAnonymous/Not logged-in
-
File Pages67 Page
-
File Size-