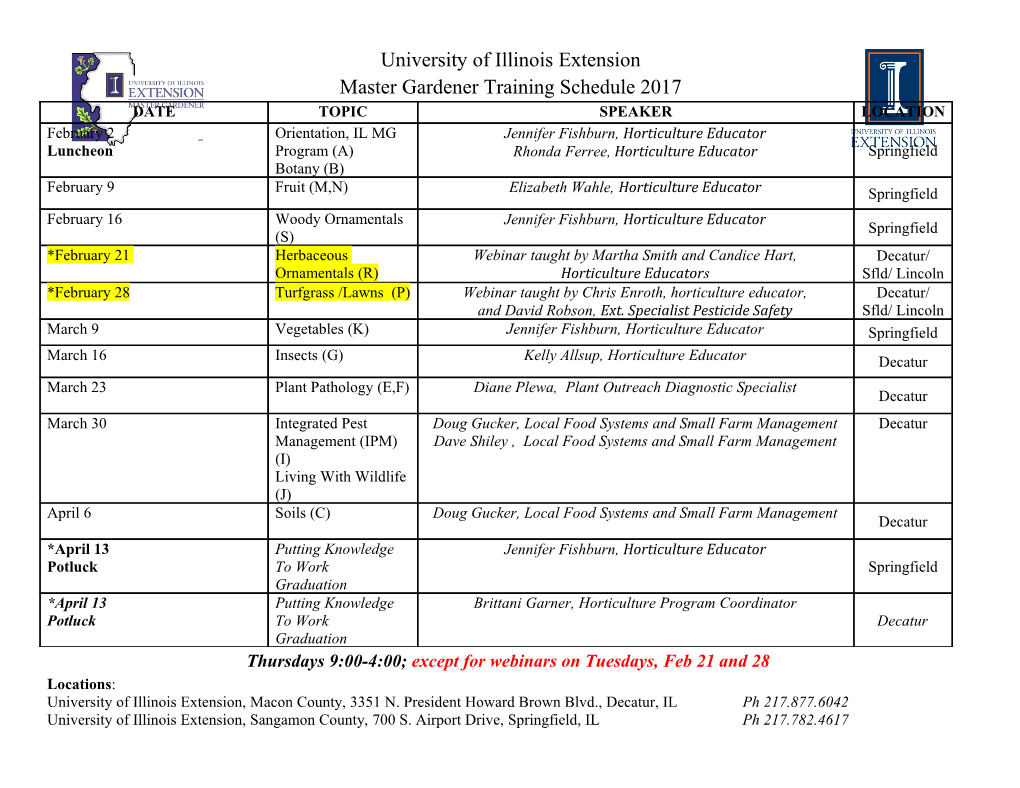
10.6 ADVANCES IN DOWNBURST MONITORING AND PREDICTION WITH GOES-16 Kenneth L. Pryor Center for Satellite Applications and Research (NOAA/NESDIS) College Park, MD 1. INTRODUCTION The three-channel GOES imager product (Pryor 2009) has been developed and The launch of Geostationary Operational experimentally implemented to assess downburst Environmental Satellite (GOES)-16 in November potential over the western United States with 2016 and subsequent activation of the Advanced improved temporal and spatial resolution. The Baseline Imager (ABI) in January 2017 has availability of the split-window channel in the resulted in the increased accessibility to high GOES-16 imager allows for the inference of spatial and temporal satellite image datasets for boundary layer moisture content. Wakimoto convective storm monitoring and nowcasting. (1985), based on his study of microbursts that The existing suite of GOES downburst prediction occurred during the Joint Airport Weather Studies algorithms employs the GOES imager and (JAWS) project, noted favorable environmental sounder to calculate potential of occurrence conditions over the western United States: (1) based on conceptual models of favorable intense solar heating of the surface and a environmental thermodynamic profiles for resulting superadiabatic surface layer; (2) a deep, downburst generation. Traditional meteorological dry-adiabatic convective boundary layer (Sorbjan satellite techniques for deep convective storm 1989) that extends upward to near the 500mb monitoring, including the water vapor – longwave level; (3) a well-mixed moisture profile with a infrared (WV – IR) brightness temperature large relative humidity gradient between the mid- difference (BTD), have recently been extended troposphere and the surface. The GOES-16 ABI by multichannel techniques for diagnosing microburst algorithm employs brightness attributes of a favorable downburst environment. temperature differences (BTD) between band 10 The WV-IR BTD has demonstrated the ability to (lower-middle level water vapor, 7.3 μm), band 14 infer convective storm structure signatures that (longwave infrared window, 11 μm), and split signify the occurrence of hazardous conditions window band 15 (12μm). Band 10 is intended to that entail large hail and severe and potentially indicate lower to middle tropospheric moisture damaging straight-line winds resulting from content and advection while band 15 indicates downbursts, and, with 2-km resolution GOES-16 surface layer moisture content. Soden and ABI data, the BTD product is able to detect fine Bretherton (1996) (SB96), in their study of the detail signatures more effectively when relationship of water vapor radiance and layer- compared to 4-km resolution GOES-13-15 average relative humidity, found a strong imagery. Accordingly, a three-channel BTD negative correlation between 7.3μm brightness algorithm incorporating split window IR channels temperature (Tb) and layer-averaged relative (11μm, 12μm) and a WV channel (6.7 μm) humidity (RH) between the 300 and 700-mb produces an output BTD that is proportional to levels. Thus, in the middle troposphere, downburst potential and supplements the decreases in Tb are associated with increases in sounder-derived Microburst Windspeed Potential RH as illustrated in Figure 4 of SB96. It follows Index (MWPI)(Pryor 2014, Pryor 2015) that is that large BTD between bands 10 and 15 imply a designed to diagnose attributes of a favorable large relative humidity gradient between the mid- downburst environment: troposphere and the surface, a condition 1) the presence of convective available potential favorable for strong convective downdraft energy (CAPE), and 2) the presence of a deep generation due to evaporational cooling in the surface-based or elevated mixed layer with a sub-cloud layer. In contrast, a positive BTD large temperature lapse rate. between bands 14 and 15 indicates a relatively moist surface layer. Thus, the GOES imager *Corresponding author address: Kenneth L. microburst risk (MBR) product is based on the Pryor, NOAA/NESDIS/STAR, 5830 University following algorithm in which the output brightness Research Ct., College Park, MD 20740; e-mail: temperature difference (BTD) is proportional to [email protected]. microburst potential: MBR (BTD) = {T15 – T10} + {T14 – T15} (1) observations that have shown positive BTD (> 0°K) can occur when water vapor exists above Where the parameter Tn represents the cloud tops in a stably stratified lower stratosphere brightness temperature observed in a particular due forced ascent from overshooting tops. Thus, imager band. The relationship between BTD and a BTD greater than zero (0°K) has been used a microburst risk in the product image is based on measure for intensity of overshooting convection. the following assumptions: (1) A deep, well- In addition, Schmetz et al. (1997) associated a mixed convective boundary layer exists in the positive BTD with a deep convective storm cloud region of interest; (2) moisture for convective top that has reached the height of the tropopause storm development is based in the mid- and lower stratosphere. A threshold BTD of > – troposphere and is advected over the region of 10°C indicates the presence of mature interest; and (3) the mid- to upper- tropospheric convective clouds, with tops approaching the layer of moisture is vertically extensive and would upper troposphere that will soon likely generate yield precipitation if provided a sufficient forcing precipitation (Mecikalski and Bedka 2006). An mechanism. The importance of the {T14 – T15} alternative application of the WV-IR BTD term derives from the contribution of a surface- technique was proposed by Pryor (2014) based based moist layer in the enhancement of on a conceptual model of a dry-air “notch” that downdraft acceleration. Srivastava (1985) noted was formulated to represent unsaturated air that that lower environmental relative humidity in the intrudes into a convective storm and interacts boundary layer, a condition typically associated with convective and stratiform precipitation with an inverted-V thermodynamic profile, has a regions, subsequently providing the energy for detrimental effect on downdraft intensity because intense downdrafts and resulting outflow winds. of the lowering of virtual temperature and the Figure 1 graphically describes the physical resultant decrease in the virtual temperature process of downburst generation within a local deficit between the evaporatively cooled storm. The presence of a dry-air “notch” on the downdraft and the surrounding environment. periphery of a convective storm suggests, on the Assuming that evaporation continues to sustain local storm scale, direct interaction of unsaturated the downdraft with approach to the surface, air with the precipitation core that results from increased water vapor content in the surface wake entrainment as described by Knupp (1989). layer will increase the environmental virtual Through numerical simulations, the author temperature and the resultant deficit, and thereby confirmed that entrainment of dry mid-level air reinforce negative buoyancy and downdraft into the downshear flank of a convective storm acceleration. fostered strong downdraft generation. This product provides a higher spatial (2 This paper presents an updated km) and temporal (5 minutes) resolution than is assessment of the imager and sounder-derived currently offered by the GOES sounder algorithms applied to GOES-16 ABI data microburst products (10 km, 60 minutes) and featuring case studies that demonstrate the thus, provides useful information to supplement effective use of cloud and moisture imagery with the sounder products in the convective storm 13-km Rapid Refresh (RAP) model data to nowcasting process. In addition, this imager simulate advanced sounder-derived products. In product provides microburst risk guidance in high addition, the sounder and imager microburst latitude regions, especially north of latitude 50°N, products will be compared and contrasted, and where existing sounder coverage is not available. this paper will outline the advantages of each Both the GOES sounder MWPI and imager product in the nowcasting process. microburst risk products were developed as predictive linear models developed in the manner 2. METHODOLOGY exemplified in Caracena and Flueck (1988). Each microburst product consists of a set of The objective of this validation effort was predictor variables that generates output of to qualitatively and quantitatively assess the expected microburst risk. performance of the GOES MWPI product and The traditional usage of the brightness imager-derived microburst products by temperature difference (BTD) between GOES employing classical statistical analysis of real- infrared channel 3 (WV, 6.5μm) and channel 4 time data. Accordingly, this effort entailed a study (IR, 11μm) is the monitoring of convective storm of downburst events over the Eastern Snake intensity by detection of overshooting River Plain (ESRP) of southeastern Idaho during thunderstorm tops. Rabin et.al. (2017) noted the 2017 convective season that was executed in a manner that emulates historic field projects 14, and 15 that specify the relative contribution such as the 1982 Joint Airport Weather Studies from each atmospheric layer to emitted radiation (JAWS) (Wakimoto 1985). GOES-16 ABI Cloud were obtained for southeastern Idaho in the and Moisture Imagery product (CMIP) datasets vicinity of INL from a Cooperative Institute for was collected for pre-convective
Details
-
File Typepdf
-
Upload Time-
-
Content LanguagesEnglish
-
Upload UserAnonymous/Not logged-in
-
File Pages13 Page
-
File Size-