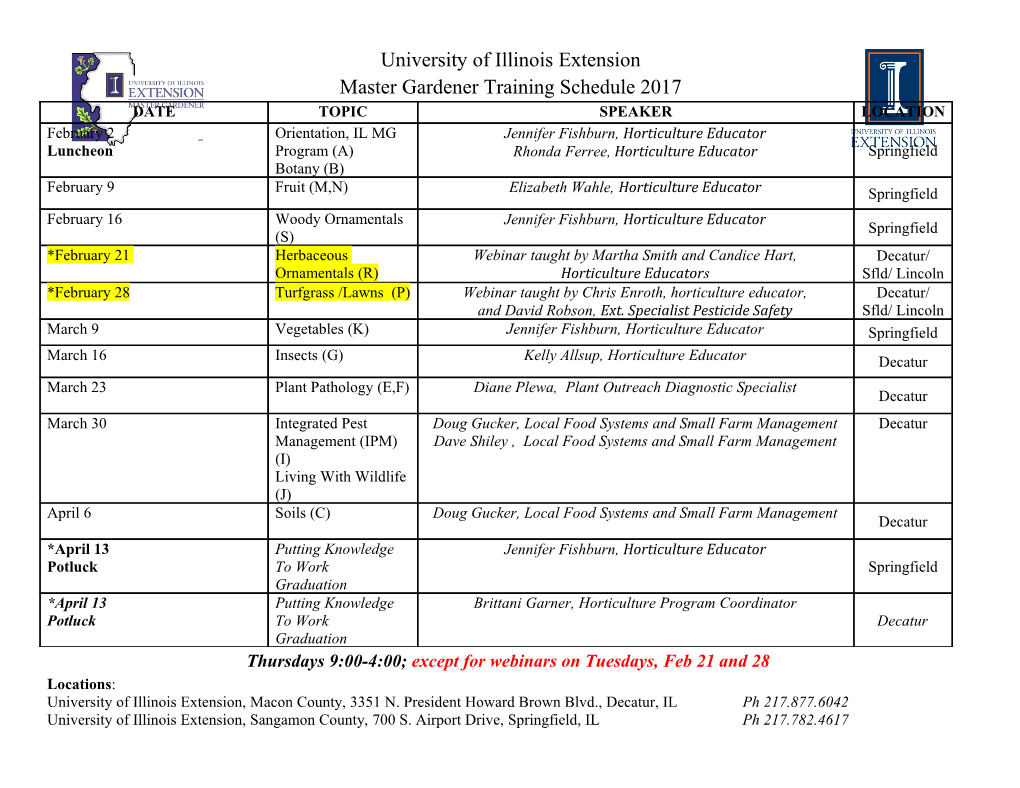
Facoltà di Scienze Matematiche, Fisiche e Naturali Dipartimento di Chimica Organica e Industriale Corso di Dottorato di Ricerca in Chimica Industriale (XXIII ciclo) Settore disciplinare: CHIM/06 CHIRAL LEWIS BASES ACTIVATION OF TRICHLOROSILYL DERIVATIVES Tutor: Prof. Maurizio Benaglia Co-Tutor: Prof. Laura Maria Raimondi Coordinatore: Prof. Dominique Roberto Tesi di Dottorato di Sergio Rossi Matr. R07820 Anno Accademico 2009-2010 To my family INDEX INTRODUCTION I CHAPTER 1 - Silicate-mediated stereoselective reactions catalyzed by chiral Lewis bases 1 1.1 Hypervalent bonding analysis 2 1.2 Achiral C-H bond formation from reduction with HSiCl3 9 1.3 Stereoselective C-H bond formation 11 1.3.1 Reactions catalyzed by N-formyl derivatives 11 1.3.2 Reactions catalyzed by chiral picolinamides 20 1.3.3 Reactions catalyzed by other chiral Lewis bases 25 1.4 Stereoselective C-C bond formation 29 1.4.1 Allyltrichlorosilane addition to C=O and C=N bonds 30 1.4.2 Aldol condensation reaction 39 1.5 Ring opening reaction of epoxides 42 1.6 Miscellaneous 44 CHAPTER 2 - New phosphoramides and phosphoramidates as catalysts in trichlorosilyl compounds-mediated reactions 47 CHAPTER 3 - Chiral phosphine oxides in present-day organocatalysis 61 CHAPTER 4 - Biheteroaromatic diphosphine oxides as catalysts in organic reactions 77 4.1 Biheteroaromatic diphosphine oxides-catalyzed stereoselective reactions 79 4.1.1 The direct aldol reaction of ketones with aromatic aldehydes 82 4.1.2 Cross-aldol reaction between two aldehydes 93 4.1.3 The direct aldol reaction of thioesters with aromatic aldehydes 95 i Index 4.1.4 Conjugate reduction and reductive aldol reaction of α,β-unsaturated ketones 104 4.2 New bisthiophene based phosphine oxides 105 4.2.1 Pathway a: synthesis and use of diarylphosphin chloride 107 4.2.2 Pathway b: synthesis and use of diarylphosphoric chloride 109 4.3 Reactivity of new bistiophene based phosphine oxides 113 4.3.1 Resolution of a racemic mixture of new phosphine oxide 116 4.4 Synthesis of bisthiophene based phosphine oxide: a novel approach 118 OUTLOOK AND PERSPECTIVE 123 CHAPTER 5 - Molecular Modeling: basic principles 125 5.1 Molecular mechanics 128 5.1.1 Force field methods 128 5.1.2 The force fields equations 130 5.1.3 Energy-miminization 132 5.1.4 Conformational analysis 134 5.2 Quantum mechanics 137 5.2.1 ab initio methods 137 5.2.2 Semi-empirical methods 139 5.3 Schrödinger® suite 141 CHAPTER 6 – Experimental section 143 6.1 Synthesis of phosphoramides 145 6.2 Synthesis of phosphoramidates 160 6.3 Synthesis of phosphine oxide: TetraMe-BITIOPO 168 6.4 Synthesis of N-acylated β-amino enones 173 6.5 Synthesis of diarylphosphin chloride 176 6.6 Synthesis of diarylphosphoric chloride 181 6.7 Synthesis of diarylphosphoric acids and esters 183 6.8 Synthesis of TetraMe-BITIOPO derivatives 190 6.9 Synthesis of TetraMe-BITIOPO derivatives: a novel approach 195 6.10 Catalysis reactions 205 6.10.1 General procedure for allylation of aldehydes with allyl trichlorosilane 205 6.10.2 General procedure for allylation of aldehydes with allyltributylstannane 205 6.10.3 Characterization of allylation products 206 6.10.4 General procedure for the synthesis of keto-imines 207 ii Index 6.10.5 General procedure for reduction of keto-imines with trichlorosilane 208 6.10.6 Synthesis of oxazines by reductive cyclization of N-acylated β-amino enones with HSiCl3 210 6.10.7 General procedure for phosphonylation of aldehydes catalyzed by phosphine oxides 212 6.10.8 Addition of silyl ketene acetals to aldehydes catalyzed by phosphine oxides 213 6.10.9 General procedure for the direct aldol condensation of ketones with aldehydes 214 6.10.10 General procedure for the direct aldol-type reactions between aldehydes 227 6.10.11 General procedure for the synthesis of thioesters 230 6.10.12 General procedure of direct aldol condensation of thioesters with aldehydes 233 6.10.13 Conjugate reduction and reductive aldol reaction of α,β-unsaturated ketones 242 6.11 Molecular Modeling: computational data 244 6.11.1 MacroModel syntax 244 6.11.2 Validation of MMFFs force field method 244 6.11.3 TetraMe-BITIOPO calculations with MacroModel 251 6.11.4 MOPAC syntax 267 6.11.5 TetraMe-BITIOPO calculations with MOPAC 267 APPENDIX 283 REFERENCES 297 LIST OF COMMON ABBREVIATIONS 311 iii Introduction “Chemists – the transformers of matter”. This sentence, taken from the book “The Periodic Table” by Primo Levi, well defines one of the major achievements of present day actual chemistry: to provide, in a controlled and economic fashion, valuable products from readily available starting materials. It is very difficult to define what chemistry actually is. Looking at the past, chemistry must be inevitably connected to alchemy, born in the II century B.C. in Egypt, where the transformations of the matter (the conversion of a metal in another one) were connected to philosophical and esoterism. Only after the introduction of the scientific method and the chemistry revolution that takes place at the end of the XVIII century, modern chemistry began its development, giving day by day a most real and precise structure for the transmutation of the matter. So, chemistry keeps evolving, new discoveries expand and amplify the interest fields and the employed method. The study of properties and structures of the constituent of the matter (atoms, molecules) and their interactions become the new chemical target: not only the properties and the structures in a static instant, but also in its transformations, that is chemical reactions. The knowledge of the atomic electronic structure is the basis of chemistry and it is responsible for the transformations of the matter; for these reasons, chemistry can be defined as a “central science”, because it has connections with many other disciplines, as natural science, physics, material science, biology and geology. Over the past 10 years, scientific research has been increasingly directed towards the study and application of new chemical processes that were environmental friendly and I Introduction less dangerous for human life. This approach, that is currently enjoying a lot of attention, is defined sustainable or, more precisely, “green chemistry”. Nowadays it is important to develop a consciousness: chemical consequences are not only limited at the properties of a given molecule or at the efficiency of a given reagent, but they interest also many other aspects about the impact of the environment where these products are employed. That’s why, it is important to consider a comprehensive vision of the process of production including not only the application of the products, but also its byproducts and wastes. Green chemistry then, implies the design of chemical products and processes that reduce or eliminate the use or generation of hazardous substances. Green chemistry is applied across the life cycle of a chemical product, including its design, manufacture, and use.[1] To do that, twelve principles are defined: 1. Prevention: it is better to prevent waste than to treat or clean up waste after it has been created; 2. Atom Economy: synthetic methods should be designed to maximize the incorporation of all materials used in the process into the final product; 3. Less hazardous chemical syntheses: wherever practicable, synthetic methods should be designed to use and generate substances that possess little or no toxicity to human health and the environment; 4. Designing Safer Chemicals: chemical products should be designed to affect their desired function while minimizing their toxicity. 5. Safer Solvents and Auxiliaries: the use of auxiliary substances (e.g., solvents, separation agents, etc.) should be made unnecessary wherever possible and innocuous when used; 6. Design for Energy Efficiency: energy requirements of chemical processes should be recognized for their environmental and economic impacts and should be minimized. 7. Use of Renewable Feedstocks: a raw material or feedstock should be renewable rather than depleting whenever technically and economically practicable; 8. Reduce Derivatives: unnecessary derivatization (use of blocking groups, protection/ deprotection, temporary modification of physical/chemical processes) should be minimized or avoided if possible, because such steps require additional reagents and can generate waste; II Introduction 9. Catalysis: the use of catalytic reagents (as selective as possible) is superior to that of stoichiometric reagents; 10. Design for Degradation: chemical products should be designed so that at the end of their function they break down into innocuous degradation products and do not persist in the environment; 11. Real-time Analysis for Pollution Prevention: analytical methodologies need to be further developed to allow for real-time, in-process monitoring and control prior to the formation of hazardous substances; 12. Inherently Safer Chemistry for Accident Prevention: substances and the form of a substance used in a chemical process should be chosen to minimize the potential for chemical accidents, including releases, explosions, and fires. It is not so simple to comply with all these principles. In our studies, we focused on the ninth principle, and in particular on the use of stereoselective organic catalysts versus that of the more traditional organometallic catalysis[2]. The organocatalytic approach fulfils many of requirements listed in the twelve principles of the green chemistry. A process based on a catalytic methodology in fact is already “green” by definition, since it is clearly stated that “catalysts are preferable to stoichiometric reagents”, because they minimize wastes and increase energy efficiency. Obviously, a catalytic transformation provides the best “atom economy”, since the stoichiometric introduction and then removal of stereocontrolling auxiliaries can be avoided, or, at least, minimized[3]. A catalyst often allows to run a reaction in milder experimental conditions, once again improving the efficiency of a process from the economic and energetic point of view. Specifically, organocatalysts may lead to the design of “safer chemicals and products”, as expected by modern synthetic chemists, also with the goal to use less hazardous solvents or reaction conditions.
Details
-
File Typepdf
-
Upload Time-
-
Content LanguagesEnglish
-
Upload UserAnonymous/Not logged-in
-
File Pages348 Page
-
File Size-