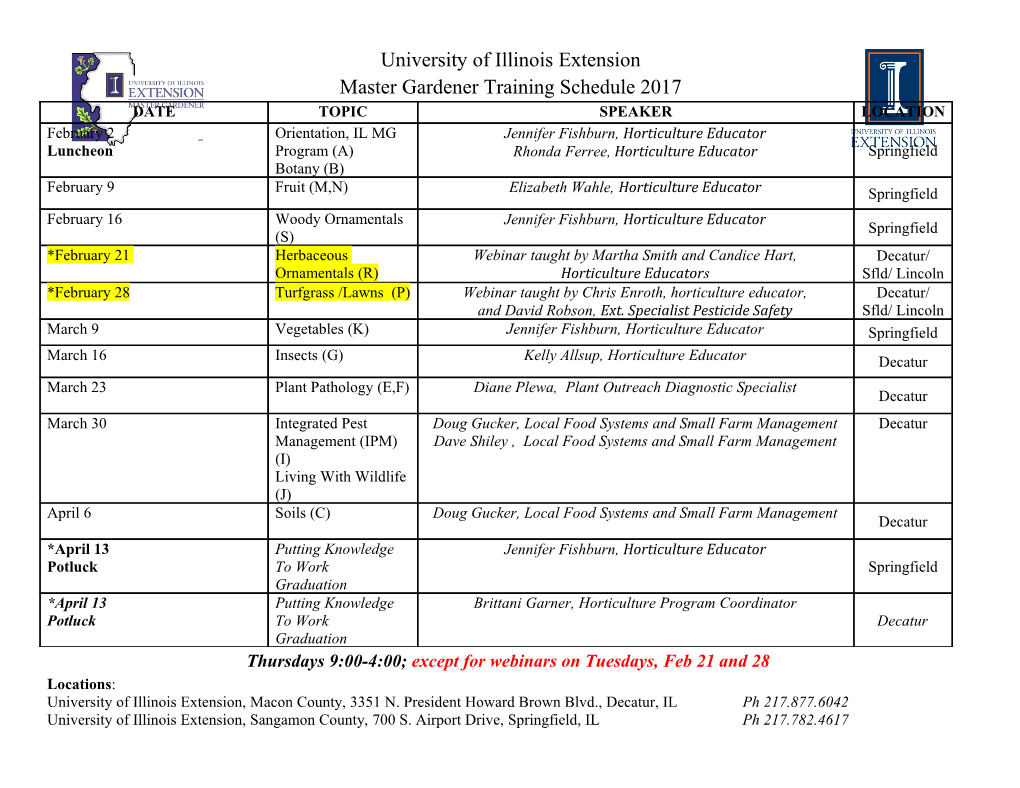
STRUCTURE AND FUNCTION OF CELL MEMBRANES1,2 MARVIN H. STROMER AND WILLIAM J. REVILLE Iowa State University3, Ames, Iowa 50010 INTRODUCTION The purpose of this presentation is to provide some backgrmnd information regarding the organization and function of cell membranes, in general, and then to focus attention on the membranes of the skeletal muscle cell. The interrelationships of the specialized membranes of the skeletal muscle cell will be discussed in order to develop a framework into which the succeeding three papers can be integrated. In attempting to ascribe a purpose or function to any biological membrane in a cell, one is rapidly forced to conclude that membranes sequester or segregate components of the cell or the cell itself from its environment and regulate what external stimuli or components may enter the cell. This is the situation for a wide variety of cells, including muscle. Mernbranes inside a cell may maintain favorable microenvironments within closed tubular or vesicular systems and prmide a structural matrix on which enzymes may be favorably oriented to interact with substrates and on which certain essential features such as ribosomes may be located to maximize their efficiency. Processes such as protein synthesized for export may be regulated or modulated by interactions with membranes. MEMBRANE MODELS a. Unit membrane model Research on membranes from a variety of sources is a very active and diverse area of investigation. The two models that attempt to explain membrane structure and components are the unit membrane or bimolecular leaflet model of Danielli-Davson-Robertson (6, 2) and the fluid mosaic model of Singer and Nicolson (28). Danielli and Davson published their original unit membrane model in 1936, and Robertson (26) has, since the 1950's, been engaged in research which supports this Journal Paper No. 5-7963 of the Iowa Agriculture and Home Economics Experiment Station, Ames. Projects No. 1795, 1796, 2025. This review supported in part by grants from the American Heart Association (No. 71-679), and the Muscular Dystrophy Associations of America. * Presented at the 27th Annual Reciprocal Meat Conference of the American Meat Science Association, 1974. 3 Muscle Biology Group, Departments of Food Technology and Animal Science cooperating 299 model. The unit membrane consists of a core of phospholipid molecules with their fatty acid tails directed inward and the polar and ionic heads directed toward the outer margins of the membrane (Fig. 1). This phospholipid core is covered on each side by a monolayer of nonlipid material, thought by many to be protein or protein-like. The work of Robertson and associates has suggested that the two covering monolayers are chemically different from each Dtner (Fig. 4) and may thereby confer an inner and an outer orientation to membranes. The unit membrane is typically observed in the electron microscope as a structure about 75 x thick and composed of two dense lines each 20 8 in thickness, which are separated by a 35 8 space of decreased electron opacity as shown in the left schematic of Fig. 2. These same dimensions are obtained from electron microscope images and X-ray diffraction patterns of membranes. Robertson and others have obtained such images fram conventional intact membranes and from myelin and have obtained corroborating evidence from lipid model systems. Of the three possible arrangements shown in Fig. 2a-c for the components of the unit membrane (namely, polar lipid heads outward with nonlipid outer covering as in a, polar lipid heads directed inward toward the core of the membrane with a nonlipid outer covering as in b, and polar heads directed inward toward a nonlipid or protein core as in c, only the first satisfies the image seen in the electron microscope. The polar ends of the lipid molecdes and the covering layer produce the dark staining zones while the nonpolar carbon chains are responsible for the lighter zone. If either of the other two pmsibilities were correct, a membrane would consist of three dense lines and two light zones as in b or a single dense band as in c rather than the tripartite structure usually seen. Multiple layers of this tripartite membrane structure would generate myelin sheaths (Fig. 3) as described by Finean and Burge (10). Permeability of unit membranes to small ions has been described as being accomplished by a carrier molecule binding to the ion and trans- porting it to the other side. Robertson, however, believes that, if an ion was bound by the covering protein coat at a particular site, an allosteric change in the protein molecule could occur and the ion could be transported across the protein layer and reside, temporarily, adjacent to the lipid core. If several ions accumulated next to the polar groups, the potential across the bilayer could increase to -400 mv. Experiments with model membrane systems indicate that such a potential is sufficient to break the lipid bilayer. This would afford a passage- way for ions to cross the remaining portion of the membrane. Three pohts regarding the present concept of the unit membrane model as shown in Fig. 4 must be stressed. First, structural features of the model are based primarily on electron microscope and X-ray diffraction analysis of intact red blood cell and myelin membranes and lipid model systems. Second, the model clearly is based on a continuous lipid bilayer covered by continuous coats of nonlipid material, presumably protein. Third, certain specialized membranes in very rare instances present structural images that can not be interpreted as typical unit membranes (16). Nevertheless, many membranes in biological systems do present this appearance. Exterior -- .. Lipoid .... interior Fig. 1. The Danielli-Davson membrane model consists of a lipid bilayer of unspecified thickness and two monolayers of protein. Note that the polar heads of the lipid molecules are directed outward and that the membrane is symmetrical. From Danielli and Davson, 1935, by permission of the author and the Wistar Press. c d 20A I." d35A d20A I rJ 75A a b C Fig. 2. The typical appearance of a unit membrane and the dhensions observedI in the electron microscope are shown on the lef't side of this diagram. Two dark 20 8 lines separated by a 35 8 space give a total membrane thickness of 75 8. Three possible arrangements of the molecular components in the unit membrane model are shown in a, b and c. Reaction of electron-dense stains with membrane components dictates that the only arrangement possible is that sham in a. From J.D. Robertson, Arch. Int. Med., Feb. 1972, Vol 129, Copyright 1972, American Medical Assn. 3 01 I Lipid I -w 170 A Protein7vvvv.m~ Fig. 3. Finean's model for the repeating unit in the myelin sheath was based on X-ray and electron microscope evidence and consists of two unit membranes which, although apparently symmetrical, give different reactions at their two surfaces to fixation and staining. From Finean, 1955 by permission of the author. Flg. 4. Schematic diagram of Robertson's concept of the unit membrane. Comparison with Fig. 1 reveals that, although polar lipid heads still point outward, the two covering layers of non-lipid are chemically different and cause the membrane to be asymmetrical. From J. D. Robertson, Arch, Int. Med., Feb. 1972, Vol. 129, Copyright 1972, American Medical Assn. b. Fluid mosaic model The second membrane model is called the fluid mosaic model (Fig. 5). Globular protein molecules are randomly dispersed in a lipid bilayer matrix. Orientatim of the protein molecules is such that the more polar portions of the molecule are directed outward toward the water interface while the less ionic portions of the molecules are found in the center of the lipid core (Fig. 6). This model is clearly based on a discontinuous distribution of protein in the membrane. Direct evidence for such a distribution comes from antibody localization and from freeze-etching experiments. Certain of the proteins known to be located in membranes were isolated and purified and used as antigens. Localization of antibodies to these antigens at the electron microscope level by Nicolson and Associates (19, 20, 21) clearly showed that the antigen was distributed randomly in the plane of the membrane. The appearance of membranes observed after the freeze etching technique (3O), parCicularly if the fracture plane occurs down the center of the lipid bilayer, is that of a fairly smooth matrjx containing a large number of usually randomly distributed particles. This is the characteristic appearance of membranes from nuclei, plasmalemma, mitochondria, bacteria and vacuoles (2, 3, 29). The size of the particle seems to be particularly characteristic of the membrane in which the particle resides. For example, in erythrory-te membranes these particles seem to be about 85 W in diameter. There also seems to be two classes of membrane proteins as reported by Singer's laboratory (27) and by Green's laboratory (24). The peri- pheral proteins are removed by mild treatments, dissociate free of lipids, and once dissociated, are relatively soluble in neutral aqueous buffers. The integral proteins require very strong treatments to free them from membranes, often remain associated with lipids after isolation, and become very insoluble if totally freed from lipid. In the fluid mosaic model, the peripheral proteins would be those only partly embedded in the lipid bilayer; the integral proteins are those more completely embedded in the lipid rnatrix and may even extend completely across the bilayer. Thermodynamic considerations of membranes and their components were the main impetus for the developnent of the fluid mosaic model of cell membranes. These considerations are centered on the hydrophobic and hydrophylic interactions known to occur either in a membrane or at its surface.
Details
-
File Typepdf
-
Upload Time-
-
Content LanguagesEnglish
-
Upload UserAnonymous/Not logged-in
-
File Pages15 Page
-
File Size-