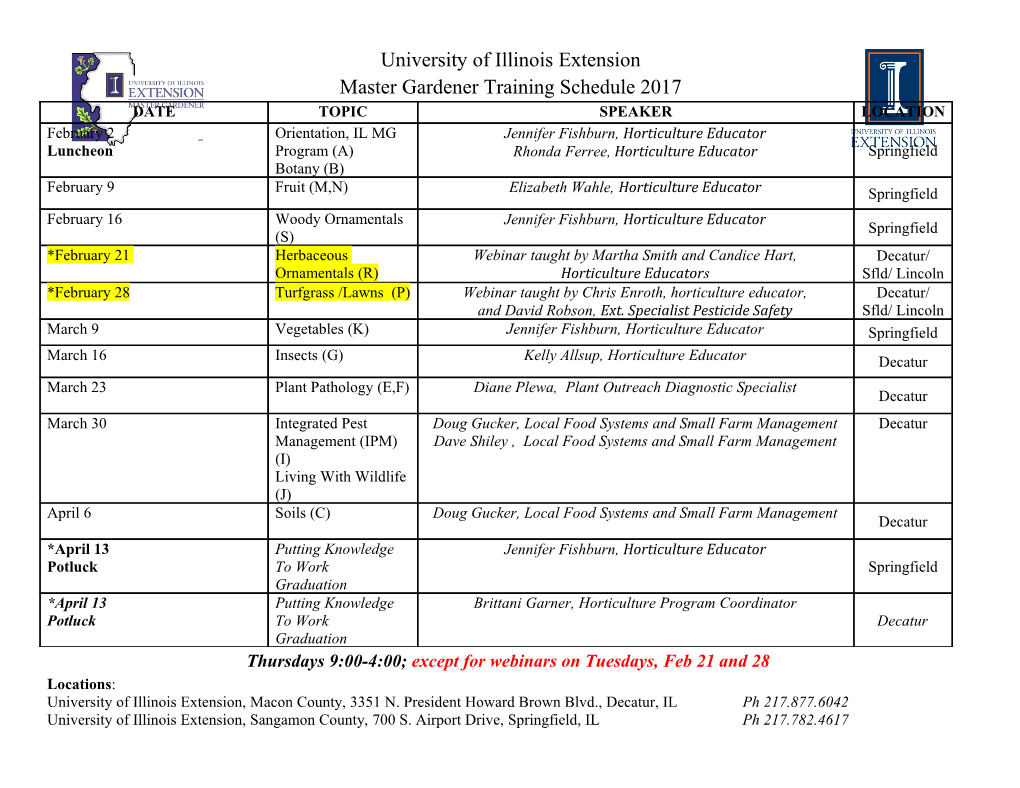
This electronic thesis or dissertation has been downloaded from Explore Bristol Research, http://research-information.bristol.ac.uk Author: Ambler, Michael T Title: Life on hold mapping the neural control of torpor General rights Access to the thesis is subject to the Creative Commons Attribution - NonCommercial-No Derivatives 4.0 International Public License. A copy of this may be found at https://creativecommons.org/licenses/by-nc-nd/4.0/legalcode This license sets out your rights and the restrictions that apply to your access to the thesis so it is important you read this before proceeding. Take down policy Some pages of this thesis may have been removed for copyright restrictions prior to having it been deposited in Explore Bristol Research. However, if you have discovered material within the thesis that you consider to be unlawful e.g. breaches of copyright (either yours or that of a third party) or any other law, including but not limited to those relating to patent, trademark, confidentiality, data protection, obscenity, defamation, libel, then please contact [email protected] and include the following information in your message: •Your contact details •Bibliographic details for the item, including a URL •An outline nature of the complaint Your claim will be investigated and, where appropriate, the item in question will be removed from public view as soon as possible. Life on hold: mapping the neural control of torpor Michael Ambler A dissertation submitted to the University of Bristol in accordance with the requirements for award of the degree of PhD in the Faculty of Life Sciences, School of Physiology, Pharmacology, and Neurosciences Submitted December 2020 Word count: 55,389 i ii Abstract Torpor is a hypothermic, hypometabolic state engaged when the availability of nutrients is insufficient to maintain homeostasis. It is observed in a wide range of animals, including the laboratory mouse, and if mimicked in humans, a synthetic torpor state could have useful clinical applications. The mechanisms that control torpor are not known. This thesis explores the neuroanatomical basis of torpor induction. The core method used a genetic approach that allowed targeted expression of transgenes in neurons that are active during torpor. First, two protocols for torpor induction in mice were validated, alongside the use of surface thermography as a proxy for internal body temperature. A method to detect torpor was developed using a moving window of the mean and standard deviation of mouse surface temperature across the 24-hour cycle. I then used the transgenic mouse model to express a fluorescent protein in neurons that were active during torpor. This identified that the dorsomedial hypothalamus (DMH) and the preoptic area of the hypothalamus (POA) increase activity during torpor. Next, a chemogenetically modified receptor was expressed in neurons that were active during torpor. Reactivation of those neurons generated a synthetic torpor state in the absence of any natural stimulus for torpor. Targeted reactivation of only the POA neurons that were active during torpor recapitulated this synthetic torpor, demonstrating that the POA contains neurons that are sufficient for torpor induction. A similar approach in the DMH demonstrated that neurons in this nucleus promote, prolong, and deepen torpor bouts in calorie restricted mice, but are neither necessary nor sufficient for torpor induction. In summary, these findings represent the first demonstration of a synthetic torpor in the mouse. They indicate that the POA is sufficient for torpor induction, and that the DMH promotes torpor but is neither necessary nor sufficient. iii iv Acknowledgements First and foremost, I would like to thank Tony Pickering and Matt Jones for humouring me when I first approached them with the idea of studying torpor, and for their ongoing support and advice since then. It is ten years since I first landed in Tony’s laboratory, and it is no coincidence that I returned to do my PhD. Thanks to the members of both groups, who provided comradeship, advice, and laughs that made the past three (and a half) years so enjoyable. Thanks to Liqun Luo at Stanford for his kind donation of the TRAP2 mice, and to the University of Bristol staff in the animal services unit, Wolfson Bioimaging Facility, and Histology Services. Thanks also to the Wellcome GW4 Clinical Academic Training scheme directors, management, and administration team, for providing the opportunity to undertake this PhD. I would also like to acknowledge the invaluable support from colleagues in Bologna. Matteo Cerri graciously shared his expertise and advised on the design of these experiments, and even loaned Timna Hitrec, whose calm and logical approach made the multiplex RNA in-situ hybridisation protocol manageable. Thanks to my family for their enthusiasm and encouragement, and to Shilpa for never letting me take any of this too seriously, and for taking up the domestic consequences when I did. v vi Author’s declaration I declare that the work in this dissertation was carried out in accordance with the requirements of the University's Regulations and Code of Practice for Research Degree Programmes and that it has not been submitted for any other academic award. Except where indicated by specific reference in the text, the work is the candidate's own work. Work done in collaboration with, or with the assistance of, others, is indicated as such. Any views expressed in the dissertation are those of the author. SIGNED: ............................................................. DATE:.......................... vii viii Contents Chapter 1 Introduction ....................................................................................... 10 1.1 Clinical Background .......................................................................................... 10 1.2 Torpor: the natural solution ............................................................................. 13 1.2.1 Physiological characteristics of torpor ...................................................... 16 1.2.2 Definition, timing, and duration of torpor ................................................ 17 1.2.3 Thermoregulation during torpor ............................................................... 19 1.2.4 Cardiovascular function during torpor ...................................................... 19 1.2.5 Metabolic rate and respiratory function during torpor ............................ 21 1.2.6 Renal function in torpor and hibernation ................................................. 24 1.2.7 Blood glucose and torpor .......................................................................... 25 1.2.8 Torpor, calorie restriction, and longevity ................................................. 27 1.3 Physiology of mammalian thermoregulation ................................................... 28 1.3.1 Afferent thermoregulation signals ............................................................ 29 1.3.2 Efferent thermoregulation signals ............................................................ 32 1.3.3 Thermoregulation and fever ..................................................................... 35 1.4 Thermoregulation, food intake and body weight ............................................ 35 1.4.1 Leptin, pro-opiomelanocortin, and alpha-melanocyte stimulating hormone .................................................................................................................. 38 1.4.2 Physiology of leptin ................................................................................... 38 ix 1.4.3 Functional anatomy of leptin .................................................................... 39 1.4.4 Neuropeptide Y and Agouti-related Peptide ............................................ 41 1.4.5 Physiology of NPY and AgRP ..................................................................... 42 1.4.6 Functional anatomy of NPY and AgRP ...................................................... 43 1.4.7 Food intake and thermoregulation: summary .......................................... 44 1.5 Thermoregulation and sleep ............................................................................ 46 1.5.1 Temperature changes during sleep .......................................................... 47 1.5.2 Functional anatomy linking sleep and thermoregulation: the preoptic hypothalamus ......................................................................................................... 49 1.5.3 Orexin, sleep, and thermoregulation ........................................................ 50 1.5.4 Sleep and adenosine ................................................................................. 52 1.5.5 Sleep and torpor ....................................................................................... 52 1.6 Mechanisms of torpor ...................................................................................... 53 1.6.1 The sympathetic nervous system and leptin in torpor ............................. 53 1.6.2 NPY, ghrelin, and torpor ........................................................................... 60 1.6.3 Adenosine, orexin, and torpor .................................................................. 62 1.6.4 Torpor and histamine ................................................................................ 64 1.6.5 Torpor and endogenous opioids ............................................................... 65 1.6.6 Functional anatomy of torpor ..................................................................
Details
-
File Typepdf
-
Upload Time-
-
Content LanguagesEnglish
-
Upload UserAnonymous/Not logged-in
-
File Pages441 Page
-
File Size-