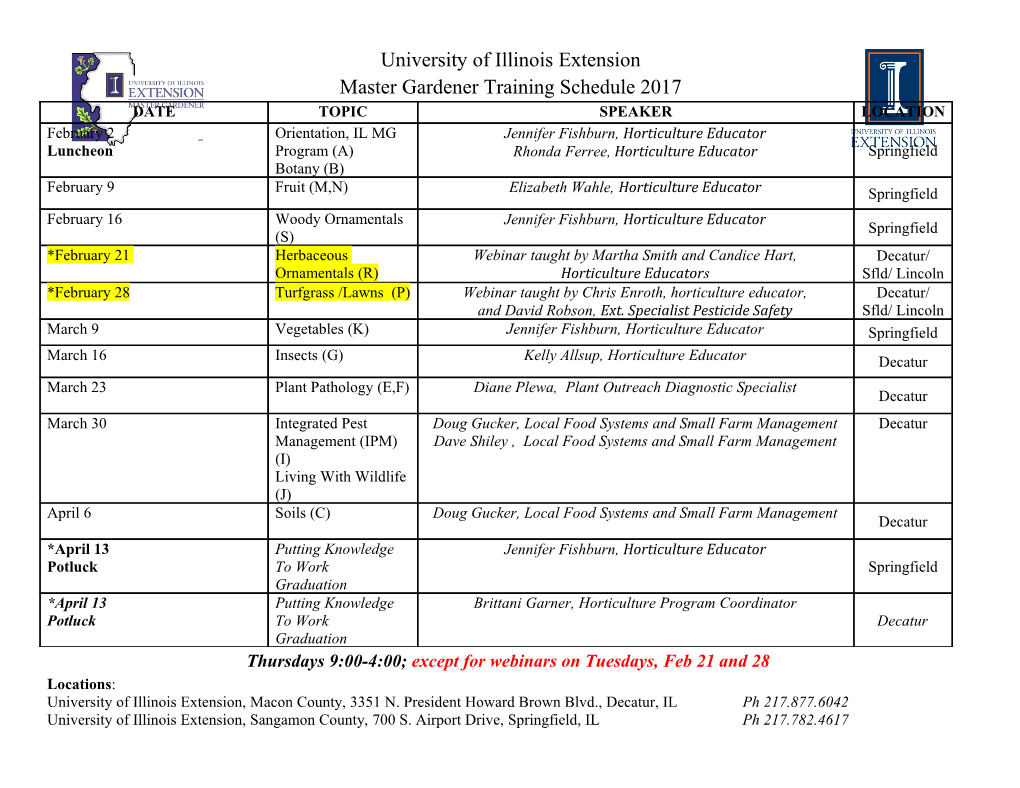
KFA-IKP(Th)-1994-12 7 March 1994 1 On determination of the large-x gluon distribution at HERA N.N. Nikolaeva,b, and B.G. Zakharovb,c aIKP(Theorie), KFA J¨ulich, 5170 J¨ulich, Germany bL. D. Landau Institute for Theoretical Physics, GSP-1, 117940, ul. Kosygina 2, Moscow V-334, Russia cInterdisciplinary Laboratory for Advanced Studies (ILAS) Miramare, I-34014 Trieste, Italy arXiv:hep-ph/9403243v2 12 Mar 1994 Abstract We discuss corrections to the Leading-LogQ2 relationships between the gluon density g(x, Q2) 2 2 2 1 and FL(x, Q ), ∂FT (x, Q )/∂ log Q in the HERA range of large x . We find that the above 2 2 2 2 quantities probe the gluon density g(x, QT,L) at QT,L = CT,LQ , with the Q -rescaling factors CT ≈ 2.2 and CL ≈ 0.42. The possibility of treating charm as an active flavour is critically re-examined. E-mail: [email protected] 1 1 Introduction. 1 2 At large x , the gluon desnity g(x, Q ) is much higher than the density of charged partons q(x, Q2), q¯(x, Q2) (hereafter x is the Bjorken variable and Q2 is the virtuality of the photon) and the photoabsorption will be dominated by interaction with the nucleon of the lightcone qq¯ Fock states of the photon via the exchange by gluons (Fig. 1) or, alternatively, by the photon-gluon ∗ 2 fusion γ g → qq¯. Consequently, the longitudinal structure function FL(x, Q ) and the slope of 2 2 the transverse structure function FT (x, Q )=2xF1(x, Q ) become direct probes of the gluon structure function G(x, Q2)= xg(x, Q2): α (Q2) F (x, Q2)= S e2 G(ξ x, Q2) , (1) L 3π f L X ∂F (x, Q2) α (Q2) T = S e2 G(ξ x, Q2) (2) ∂ log(Q2) 3π f T X 2 In Eqs. (1,2) αS(Q ) is the running strong coupling, ef is the quark charge in units of the electron charge and the summation goes over the active flavours f. The suggestion of FL as a probe (partonometer) of the gluon density is due to Dokshitzer [1], Eq. (1) was elaborated in [2]. Eq. (2) readily follows from formulas (6.37) and (6.34) of the Roberts’ textbook [3] (see also [4]). Both equations were derived in the Leading-LogQ2 approximation (LLQA), the x-rescaling 1 factors ξT,L ≈ 2 [2-4]. The emergence of the gluon-dominated scaling violations at large x was clearly demonstrated in the recent QCD-evolution analysis of the NMC structure functions [5]. The obvious advantage of Eqs. (1,2) is that one does not need solving the coupled QCD-evolution equations for the gluon and (anti)quark densities. As a byproduct of our analysis [6] of determination of the BFKL pomerons intercept from 2 2 2 FL(x, Q ), ∂FT (x, Q )/∂ log Q we have noticed the potentially important corrections to the LLQA. One obvious issue is which Q2 is sufficiently large for charm to be treated as an active 2 2 2 10 flavor, because for the (u,d,s) active flavours ef = 3 compared to ef = 9 if charm also were P P an active flavour. Our analysis [6] suggests that it is premature to speak of Nf = 4 active flavours 2 2 2 2 2 unless Q >∼ (100 − 200)GeV . In [6] we also noticed that FL(x, Q ) and ∂FT (x, Q )/∂ log Q 2 2 probe the gluon structure function G(x, QT,L) at different values of QT,L, which are both different from Q2. Because of importance of determination of the gluon structure function, which is a fundamental quantity in the QCD parton model, in this paper we present an update of Eqs. (1,2) 2 which are valid also in the BFKL (Balitzkii-Fadin-Kuraev-Lipatov [7]) regime, i.e., beyond the LLQA. 1 At large x , deep inelastic scattering can be viewed as an interaction with the target nucleon of the lightcone qq¯ Fock states of the photon via the exchange by gluons (Fig.1). The principal 2 quantities are the probability densities |ΨT,L(z,r)| for the qq¯ Fock states with the transverse size ~r and the fraction z of photon’s lightcome momentum carried by the (anti)quark, and σ(x, r) - the total cross section of interaction of the qq¯ colour dipole of transverse size r with the nucleon target [8,9]. This dipole cross section satisfies the generalized BFKL equation derived in [9,10] and is related to the differential density of gluons by the equation [9,11,12] 2~ ~ 2 π 2 d k 4[1 − exp(ik ~r)] ∂G(xg,k ) σ(x, r)= αS(r)r 2 · 2 2 2 . (3) 3 Z k k r ∂ log k The wave functions of the qq¯ Fock states of the (T) transverse and (L) longitudinal photon were derived in [8] and read Nf ¯ 6α |Ψ (z,r)|2 = e2 |Ψ(ff)(z,r)|2 = em e2 {[z2 + (1 − z)2]ε2K (εr)2 + m2 K (εr)2} , (4) T f T (2π)2 f 1 f 0 X X1 Nf ¯ 6α |Ψ (z,r)|2 = e2 |Ψ(ff)(z,r)|2 = em 4e2 Q2 z2(1 − z)2K (εr)2 , (5) L f T (2π)2 f 0 X X1 2 2 2 where Kν(x) are the modified Bessel functions, ε = z(1 − z)Q + mf and mf is the quark mass. The resulting photoabsorption cross sections are equal to ([8], see also [13]) 1 1 2~ ∗ 2 2 2 2αem 2 d k σT (γ N, x, Q )= dz d ~r |ΨT (z,r)| σ(x, r)= ef dz 0 π 0 k4 Z Z Xf Z Z 2 2 2 ~ 2 2 2 2 ~ ~ 2 2 d ~κ [z + (1 − z) ]k + mf [z + (1 − z) ]k(k + ~κ)+ mf ∂G(xg,k ) 2 − αS(q ) , (6) ~κ2 + ε2 ~ 2 2 ~ 2 2 ∂ log k2 Z k + ε (k + ~κ) + ε 1 1 2~ ∗ 2 2 2 2αem 2 2 2 2 d k σL(γ N, x, Q )= dz d ~r |ΨL(z,r)| σ(x, r)= ef dz 4Q z (1 − z) 0 π 0 k4 Z Z Xf Z Z 2 2 d ~κ 1 1 ∂G(xg,k ) 2 − αS(q ) , (7) 2 2 ~ 2 2 ~ ~ 2 2 Z ~κ + ε (k + ε k(k + ~κ)+ ε ) ∂ log k 2 Here the running coupling αS(q ) enters the integrand at the largest relevant virtuality, q2 = max{ε2 + κ2,k2} , (8) 3 and the density of gluons enters at M 2 x = x(1 + t ) (9) g Q2 ∗ where Mt is the transverse mass of the produced qq¯ pair in the photon-gluon fusion γ g → qq¯: m2 + ~κ2 m2 +(~κ + ~k)2 M 2 = f + f . (10) t 1 − z z The flavour and Q2 dependence of structure functions is concentrated in wave functions (4,5), whereas the dipole cross section σ(x, r) (the differential gluon density ∂G(x, k2)/∂ log k2 in the momentum representation) is universal for all flavours. The important virtue of the (~r, z) rep- 2 resentation in (6,7) is the factorization of integrands as |ΨT,L(z,r)| σ(x, r), which corresponds to the exact diagonalization of the diffraction scattering matrix in the (~r, z)-representation. Furthermore, the above dipole-cross section representation (6,7) and wave functions (4,5) are valid also in the BFKL regime, i.e., beyond the LLQA, and allow an easy incorporation of 1 the unitarity (absorption) corrections at large x [9,11], with allowance for which Eq. (3) must rather be regarded as an operational definition of the gluon density beyond the LLQA. The x (energy) dependence of the dipole cross section σ(x, r) comes from the higher qqg¯ 1...gn Fock 1 states of the photon, i.e., from the QCD evolution effects, described at large x by the gen- eralized BFKL equation [9,10]. The structure functions are given by the familar equation 2 2 2 FT,L(x, Q ) =(Q /4παem)σT,L(x, Q ). We advocate using FL and FT = 2xF1, which have simpler theoretical interpretation compared to F2 = FT + FL which mixes interactions of the transverse and longitudinal photons. For the sake of completeness, we notice that, in the Born approximation the differential gluon density is related to the two-body formfactor of the nucleon hN| exp(i~k1~r1 + i~k2~r2)|Ni by the equation [12] ∂G(x, k2) 4 = α (k2)(1 −hN| exp(i~k(~r − ~r )|Ni , (11) ∂ log k2 π S 1 2 and the limiting form of Eqs. (6,7) derived in [8] is obtained. 2 2 The leading contribution comes from values of Mt ∼ Q , so that xg ∼ 2x. Strictly speaking, 1 the x-rescaling factors can not be determined within the Leading-Log x approximation. From the 2 practical point of view, when analysing FL and ∂FT /∂ log Q , it is sufficient to use xg = ξT,Lx with ξT,L ≈ 2 as determined in [2-4]. In this communication we concentrate on corrections to the LLQA. 4 2 Active flavours and the onset of LLQA The ratio σ(x, r)/r2 is a smooth function of r. Similarly, ∂G(x, k2)/∂ log k2 is a smooth function of k2. Consequently, it is convenient to use the representations 2 1 dr σ(x, r) ¯ F (x, Q2)= e2 Φ(ff)(Q2,r2) , (12) T π3 r2 r2 f T Z X 2 2 1 dr σ(x, r) 2 (ff¯) 2 2 FL(x, Q )= 3 2 2 ef WL (Q ,r ) π Z r r 2 2 X 2 α (Q ) dk ¯ dG(ξ x, k ) = S e2 Θ(ff)(Q2,k2) L , (13) 3π f k2 L d log k2 X Z 2 2 ∂FT (x, Q ) 1 dr σ(x, r) 2 (ff¯) 2 2 2 = 3 2 2 ef WT (Q ,r ) (14) ∂ log Q π Z r r 2 2 X 2 α (Q ) dk ¯ dG(ξ x, k ) = S e2 Θ(ff)(Q2,k2) T , (15) 3π f k2 T d log k2 X Z (ff¯) (ff¯) where the weight functions ΦT and WT,L are defined by 1 (ff¯) 2 2 2 2 4 (ff¯) 2 ΦT (Q ,r )=(π /4αem) dz Q r |ΨT (z,r)| , (16) Z0 1 (ff¯) 2 2 2 2 4 (ff¯) 2 WL (Q ,r )=(π /4αem) dz Q r |ΨL (z,r)| , (17) Z0 (ff¯) 2 2 ¯ ∂Φ (Q ,r ) W (ff)(Q2,r2)= T , (18) T ∂ log Q2 and the kernels ΘT,L are given by 2 2 2 2 dr αS(q ) 4[1 − J0(kr)] 2 2 ΘT,L(Q ,k )= 2 2 2 WT,L(Q ,r ) .
Details
-
File Typepdf
-
Upload Time-
-
Content LanguagesEnglish
-
Upload UserAnonymous/Not logged-in
-
File Pages16 Page
-
File Size-