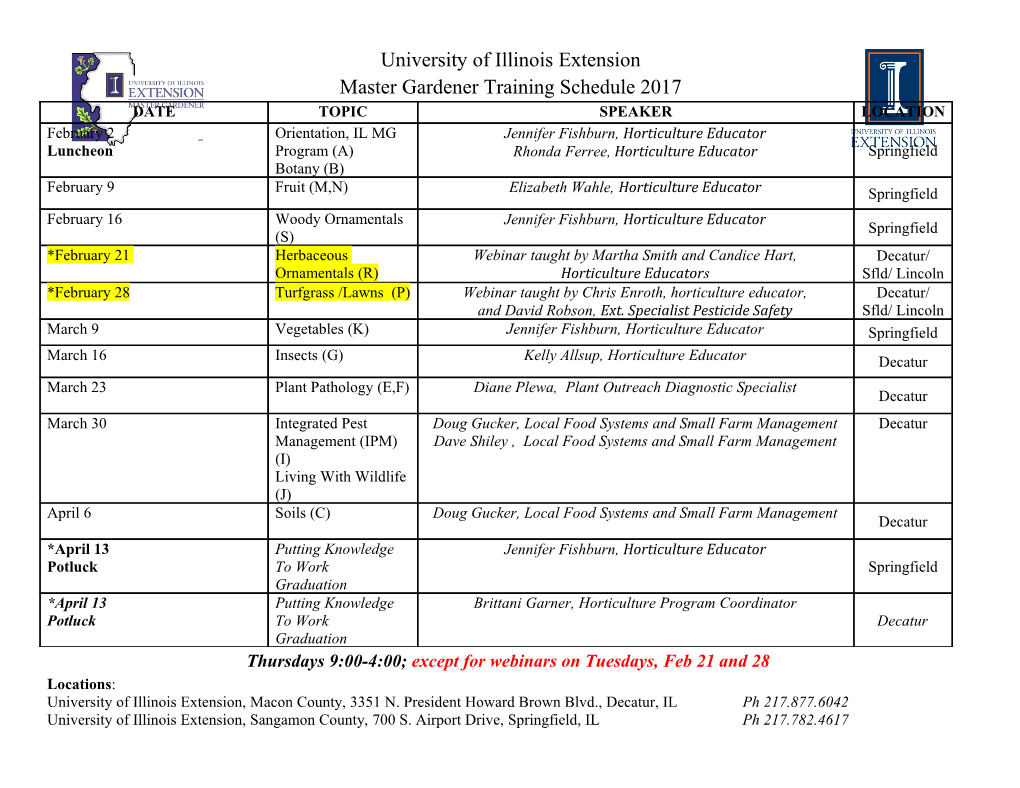
View metadata, citation and similar papers at core.ac.uk brought to you by CORE provided by South East Academic Libraries System (SEALS) APPLICATIONS OF THE BAYLIS-HILLMAN REACTION IN THE SYNTHESIS OF COUMARIN DERIVATIVES A thesis submitted in fulfilment of the requirements for the degree DOCTOR OF PHILOSOPHY of RHODES UNIVERSITY by MUSILIYU AYODELE MUSA M.Sc (Lagos) Department of Chemistry Rhodes University Grahamstown January 2002 ABSTRACT The reaction of specially prepared salicylaldehyde benzyl ethers with the activated alkenes, methyl acrylate or acrylonitrile, in the presence of the catalyst, DABCO, has afforded Baylis-Hillman products, which have been subjected to conjugate addition with either piperidine or benzylamine. Hydrogenolysis of these conjugate addition products in the presence of a palladium-on-carbon catalyst has been shown to afford the corresponding 3-substituted coumarins, while treatment of O-benzylated Baylis- Hillman adducts with HCl or HI afforded the corresponding 3-(halomethyl)coumarins directly, in up to 94%. The 3-(halomethyl)coumarins have also been obtained in excellent yields (up to 98%) and even more conveniently, by treating the unprotected Baylis-Hillman products with HCl in a mixture of AcOH and Ac2O, obtained from tert-butyl acrylate and various salicylaldehydes. The generality of an established route to the synthesis of coumarins via an intramolecular Baylis-Hillman reaction, involving the use of salicylaldehyde acrylate esters in the presence of DABCO, has also been demonstrated. Reactions between the 3-(halomethyl)coumarins and various nitrogen and carbon nucleophiles have been shown to proceed with a high degree of regioselectivity at the exocyclic allylic centre to afford 3-substituted coumarin products. The electron- impact mass spectra of selected coumarin derivatives have been investigated using high-resolution and B/E linked scan data. Fragmentation pathways have been proposed and fragmentation modes associated with different coumarin-containing analogues have been compared. A series of coumarin-containing analogues of ritonavir (a clinically useful HIV-1 protease inhibitor) have been prepared and characterized. The synthetic approach has involved the coupling of coumarin derivatives with a hydroxyethylene dipeptide isostere to afford ritonavir analogues containing coumarin termini. An interactive docking procedure has been used to explore the docking of ritonavir and a coumarin- containing analogue into the enzyme active site. TABLE OF CONTENTS Page 1. INTRODUCTION 1.1 Distribution, structure and nomenclature of coumarins 1 1.2 Naturally occurring coumarins 2 1.2.1 7-Oxygenated coumarins 2 1.2.2 Dioxygenated coumarins 3 1.2.2.1 6,7-Dioxygenated coumarins 3 1.2.2.2 5,7-Dioxygenated coumarins 5 1.2.2.3 7,8-Dioxygenated coumarins 6 1.2.3 Trioxygenated Coumarins 7 1.2.3.1 6,7,8-Trioxygenated coumarins 7 1.2.3.2 5,6,7-Trioxygenated coumarins 8 1.2.3.3 5,7,8-Trioxygenated coumarins 9 1.2.4 Tetra-oxygenated coumarins 10 1.2.5 3-Substituted coumarins 10 1.2.6 4-Substituted coumarins 11 1.3 Biologically active coumarins 12 1.3.1 Anticoagulant compounds 12 1.3.2 Antibiotics and Antibacterial coumarins 14 1.3.3 Biologically active fluorescent and photostable coumarins 15 1.3.4 Potential antipsychotic compounds 17 1.3.5 Anti-HIV compounds 17 1.4 Methods of coumarin synthesis 23 1.4.1 Knoevenagel condensation 23 1.4.2 Wittig reaction 25 1.4.3 Perkin reaction 27 1.4.4 Pechmann reaction 28 1.4.5 Michael reaction 31 1.4.6 Claisen rearrangement 33 1.4.7 Palladium-catalysed addition 35 1.5 Previous work in the group and aims of the present 37 investigation 2. DISCUSSION 2.1. Application of the Baylis-Hillman reaction in the synthesis 40 of coumarin derivatives 2.1.1. The Baylis-Hillman reaction 40 2.1.2. Preparation of salicylaldehyde benzyl ethers 44 2.1.3. Synthesis of Baylis-Hillman products 45 2.1.4. Baylis-Hillman reactions between salicylaldehydes and 53 tert-butyl acrylate 2.1.5. Conjugate addition to protected Baylis-Hillman products 57 2.1.6. Synthesis of 3-substituted coumarin derivatives 62 2.1.6.1. Reductive deprotection and cyclization of conjugate 62 addition products. 2.1.6.2. Acid-catalyzed deprotection and cyclization of the O- 71 benzylated Baylis-Hillman products. 2.1.6.3. Synthesis of coumarin derivatives via direct cyclization 79 of unprotected Baylis-Hillman products. 2.1.7. Intramolecular Baylis-Hillman reactions 83 2.2. Regioselectivity of nucleophilic attack on various 87 coumarin substrates. 2.2.1. Reaction with nitrogen nucleophiles 89 2.2.2. Reaction with enolate nucleophiles 90 2.2.3. Reaction with Grignard reagents 95 2.3. Mass spectrometric studies of 3-substituted coumarins 97 2.4 Potential HIV-1 protease inhibitors 104 2.4.1. Synthesis of the diamine “backbone” 107 2.4.2. Synthesis of coumarin-containing ritonavir analogues 107 2.4.2. Molecular modelling studies of a ritonavir analogue 110 2.5. Conclusions 118 3. EXPERIMENTAL 3.1 General 120 3.2 Preparative procedures 121 3.2.1. Preparation of salicyaldehyde benzyl ethers 121 3.2.2. Preparation of Baylis-Hillman products 124 3.2.2.1. Optimization studies 124 3.2.2.2. Preparation of O-benzylated Baylis-Hillman products 126 3.2.2.3. Baylis-Hillman reactions of salicylaldeydes and 131 tert-butyl acrylate 3.2.3. Reactions of nitrogen nucleophiles with Baylis-Hillman products 134 3.2.3.1. Conjugate addition reactions with benzylamine 134 3.2.3.2. Conjugate addition reactions with piperidine 138 3.2.4. Synthesis of coumarins via conjugate addition products 142 3.2.5 Miscellaneous reactions 148 3.2.6. Synthesis of coumarins via acid-catalysed reactions of O- 150 benzylated Baylis-Hillman products 3.2.7. Synthesis of coumarins via intramolecular Baylis-Hillman 159 reactions 3.2.7.1. Preparation of salicylaldehyde acrylate esters 159 3.2.7.2. Preparation of coumarin-derived quaternary DABCO salts. 162 3.3 Nucleophilic substitution reactions of coumarin derivatives 164 3.4 Potential HIV-1 protease inhibitors 173 3.4.1. Preparation of hydroxyethylene dipeptide isostere 173 3.4.2 Preparation of coumarin-containing ritonavir analogues 176 3.5 High-resolution mass spectrometric data 180 4. REFERENCES ACKNOWLEDGEMENTS I would like to thank my supervisor, Prof Perry Kaye for all his help, guidance and assistance throughout the course of the project. It has been a great pleasure and wonderful learning experience to work under his supervision. His support and encouragement are highly appreciated. I will also like to thank Mr A. Sonemann for collecting low-resolution MS data, Dr. P. Boshoff of Cape Technikon Mass Spectrometry Unit for collecting high-resolution MS data and Rhodes Technical Staff for all their assistance. I would also like to thank my colleagues in the Chemistry Department for their help and moral support throughout my stay at Rhodes University. Thanks to Kevin Lobb for assisting with computer-related problems including computer modelling. I delicate this research work to the Almighty God, who strengthened me during the course of this work. Very special thanks to my wife and daughter, Mrs Kebeh Musa and Damilola Musa for their encouragement, understanding and love. My sincere gratitude goes to my mother and sisters for their moral and financial support. To Prof S.Radloff and Dr Tim Radloff, Pastor John Sloane and Mrs Debbie Sloane, Mrs Cornela and Mr Philip and to the entire Assembly of God Church, thanks a million for your prayers and love. 1. INTRODUCTION 1.1 Distribution, structure and nomenclature of coumarins Coumarin and many of its derivatives occur naturally. The parent heterocycle, coumarin (Figure 1) was first isolated in 1920 by Vogel1 from the fruit of Dipteryx odorata Wild. The common name, coumarin, comes from another plant Coumarouna odorate, in which it is found; the systematic name is 2H-1-benzopyran-2-one. 2, 3. 5 4 6 3 7 2 O O 8 1 Figure 1. Structure of coumarin and atom numbering Many compounds, which contain the coumarin moiety, exhibit useful and diverse biological activity and, in recent years, there has been a growing interest in their synthesis.4 Some of these coumarin derivatives have been found to be useful in photochemotherapy, antitumor and anti-HIV therapy,5,6 and as CNS-stimulants,7 antibacterials,8, 9 anticoagulants10, 11 and dyes.12 Coumarins are widely distributed throughout the plant kingdom, with the vast majority carrying an oxygen substituent at the C-7 position. 7-Hydroxycoumarin (umbelliferone) is often regarded as the parent (in a structural and biogenetic sense) of a large number of structurally more complex coumarins.2 Coumarins occur as secondary metabolites in the seeds, roots and leaves of many plant species; their function is far from clear, although suggestions include plant growth regulators, fungistats, bacteriostats and , even, waste products.13 Synthetic coumarins are widely used as aroma chemicals because of their odour strength, tenacity, stability to alkali and relatively cheap price; applications include use as a sweetener and fixative (in perfume); fragrance enhancers (for natural essential oils); blenders (in soaps and detergents); aroma enhancers (in tobacco); and for imparting pleasant odours to industrial products.3 1 Introduction 1.2. Naturally occurring coumarins Most reviews classify coumarins according to whether particular compounds are simple coumarins or derivatives of linear or angular furanocoumarins or pyranocoumarins. Murray et al.2 used a biogenetically related approach based upon the number of nuclear oxygen atoms in classifying coumarin-containing compounds – an approach which will be used in this survey of naturally occurring coumarins. Unfortunately, many natural coumarins have been assigned botanically derived names, with many ending with the suffixes, “–ol” or “–one”. 1.2.1. 7-Oxygenated coumarins Two new coumarins have been isolated from the root of Peucedanum ostruthium(L) Koch, which has been used since ancient times in folk medicine against various diseases.
Details
-
File Typepdf
-
Upload Time-
-
Content LanguagesEnglish
-
Upload UserAnonymous/Not logged-in
-
File Pages218 Page
-
File Size-