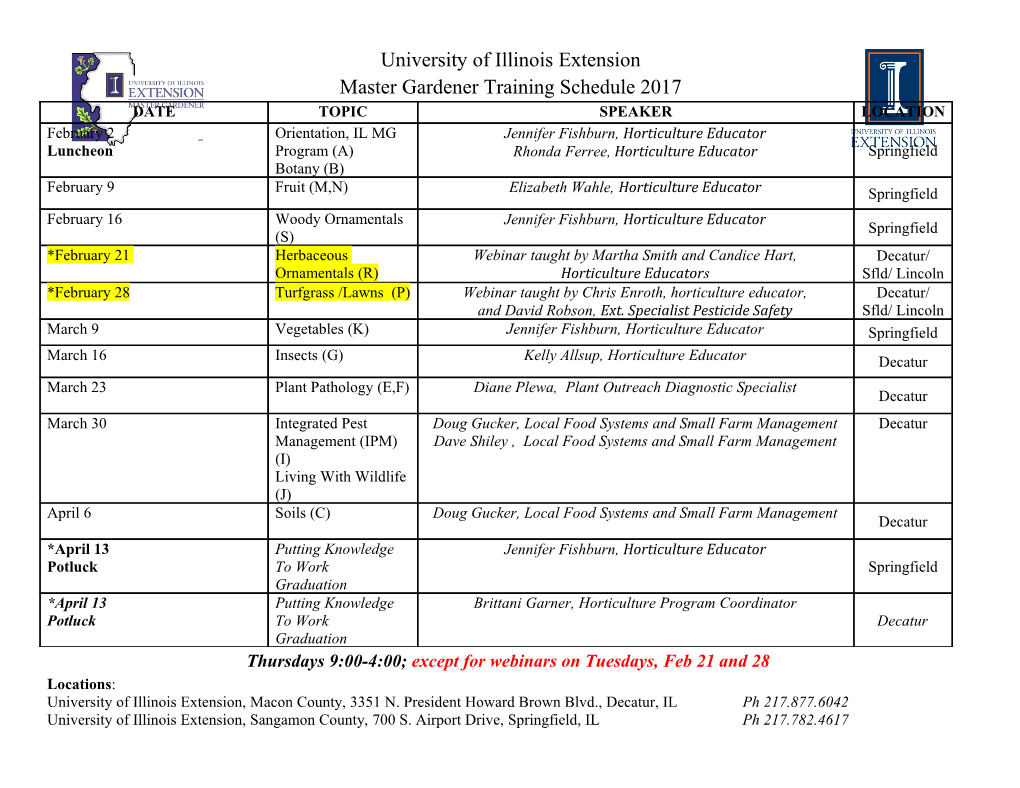
Exploration of phase modulations of non-transiting exoplanets with TESS data Neil McCulloch Department of Physics, University of Warwick, Coventry CV4 7AL, United Kingdom March 1, 2021 This report aims to explore the possibility of observing phase modulations from a non transiting exoplanets using photometry from the transiting exoplanet survey satellite. By calculating or constraining parameters of orbital systems, namely: inclination, planet radius, planet equilibrium temperature and albedo, the expected amplitude of phase modulations can be estimated and fit to the observed photometric data. Planet radius was estimated as (Rp = 1.3 ± 0.2) RJ , inclination was estimated as (i = 77.5 ± 0.2) ° and geometric albedo was constrained to (Ag = 0.37 ± 0.07). By constraining albedo and calculating planet equilibrium temperature, a basic insight into non-transiting exoplanet atmospheres was gained. 1 Introduction Data from from RV surveys implies 5- 10% of solar systems with sun-like host As of 06/01/20, there are 4104 confirmed stars contain massive planets and up to detection's [1] of planets external to our so- 30% contain rocky planets [3]. Extrapolat- lar system discovered by various methods, ing these numbers to incorporate all stars notably: transit photometry, radial veloc- in the Milky Way implies our galaxy could ity (RV) and microlensing. The majority of contain billions of planets, many of which exoplanets (¡ 75%) are discovered by ob- could orbit in the habitable zone (HZ) [3]. serving a planetary transit across its host Currently „ 15 confirmed exoplanets fit star. The resulting dip in the light curve of this criteria. the star provides information about orbital period (P ) and planet radius (Rp) [2]. 1 1.1 Discovery History 1.3 Current Search status Early search efforts relied on large varia- The first exoplanet signals were detected tions in stellar RV measurements, corre- in 1989 but it wasn't until 1992 that an sponding to massive planets orbiting close exoplanet detection was confirmed. Sub- to their host star. This limitation led to sequent discoveries via RV measurements, an early abundance of hot Jupiters discov- including the 2019 Nobel prize winners ered. However, improving camera qual- in 1995, prompted a rapid expansion in ity and precision radiotelescopes have sug- the field. Throughout the 2000s discover- gested a more diverse exoplanet distribu- ies steadily increased via numerous ground tion. Kepler categorises its candidates by based search projects, notably: HARPS, radius as follows, shown in brackets are the HAT and WASP. More recently space percentage of each discovered [1]: based telescopes including: CoRoT, Kepler and TESS have been responsible for the 1. Earths, RC ă 1:25 (20%) majority of discoveries. 2. Super-Earths, 1:25 ¤ RC ă 2 (30%) 3. Neptunes, 2 ¤ RC ă 6 (40%) 1.2 Variety 4. Jupiters, 6 ¤ RC ă 15 (7%) 5. Large Jupiters, 16 ¤ R ă 25 (3%) As discoveries have become more frequent, C the diverse nature of host stars, orbits and Kepler was the first survey to uncover the the planets themselves has come to light. large quantity (¡ 75%) of planets with Main-sequence (MS), low metallicity, gi- radii from (1:25 RC - 6 RC) orbiting sun- ant, white dwarfs, red dwarfs and pulsars like stars. Little is understood about these have all been found to harbour exoplan- super-Earth's/mini-Neptune's as they do ets. Rocky planets, as well as hot and ice not exist in our solar system. giants have been found, with masses es- Target stars selected for surveys are of- timated via RV measurements and atmo- ten purposefully skewed to maximise the sphere's probed using spectroscopic tech- detection of a specific kind of planet, for niques [4]. A variety of orbits have been ob- example, Kepler observed stars likely to served, including many early observations harbour an Earth sized planet, therefore, of tidally locked hot Jupiters (ă 0:5AU or- most Kepler host stars are sun-like (K,G,F) bit) as well as larger and often eccentric dwarfs [6], [7]. A positive correlation be- orbits [5]. tween metallicity of the star and the proba- 2 bility of an orbiting planet for MS stars was times larger than Kepler's observation field. subsequently uncovered from Kepler data The primary science objective is the detec- [8]. tion of hundreds of planets smaller than Due to the nature of exoplanet surveys, Neptune whilst maximising the possibility most candidates have small orbital periods future atmospheric reconnaissance [15]. (ă 50 days) and therefore small semi-major TESS targets a pre-selected catalogue axes (ă 0:5AU) [9], [7], [10]. Unlike most of [16] of stars at a 2 minute cadence, most our solar system, many of these orbits are of which are in the spectral range F5 - M5, eccentric, however, when multiplicity (15% limiting the survey to bright dwarfs in an of host stars [1]) is taken into account, ev- effort to detect the strongest signals. This idence suggests these two factors are anti- range will include a significant proportion correlated [11]. of abundant M dwarfs not explored by Ke- Currently it is difficult to reach the pre- pler. Like Kepler, TESS will mostly de- cision required to detect atmospheric con- tect short period planets (P „ 10 days) ditions with only limited successes [12], al- as these are much easier to observe as the though, future exoplanet reconnaissance is number of observable transits scales with expected to improve with the launch of up- P ´5{3 [17], [18]. Planets with longer peri- coming missions: PLATO [13] (character- ods will be detected in the continuous view- izing rocky planets) in 2026 and the James ing zone of TESS' ecliptic poles. These re- Web Space Telescope (JWST) [14] in 2021 gions of the sky correspond with the regions (studying planet atmospheres). which JWST can monitor at any time, en- abling easy follow up observations. Cur- 1.4 TESS rently, TESS has over 1700 project can- didates and 42 confirmed exoplanets [1]. Launched in April 2018, the transiting ex- TESS has four identical cameras, each with oplanet survey satellite (TESS) [15] will complete a two year all sky survey expect- ing to discover thousands of exoplanets. TESS will split up the northern and south- ern hemispheres into 13 overlapping sec- tors, shown in Fig. 1, which it will contin- Figure 1: (a) The FOV of the four TESS cameras. (b) Schematic of TESS sectors projected onto the uously monitor for 27.4 days each, in total sky, monitored for 27.4 days each. (c) Total ob- observing more than 200; 000 of the bright- servation duration for regions of the sky, note the JWST continuous viewing zone, the region of the est and closest MS stars over an area 400 sky why JWST will be able to monitor at any time. 3 has a 24 ˆ 24° field of view (FOV). Each Parameters Orbital camera has four CCDs with a pixel scale P pdaysq period of 21 arcseconds per pixel. TESS uses a a pAUq semi-major axis e eccentricity single wide-band filter centred on the I c i p°q inclination band, shown in Fig. 2. The band de- α pradq phase angle 0 pdaysq epoch tects photons from the red visible and infra- red spectrum with a large width to reduce Planetary MJ mass of Jupiter photon-counting noise. TESS performs re- Mp pMJ q planet mass markably well when observing bright stars, RJ radius of Jupiter Rp pRJ q planet radius m 8, reaching a standard deviation be- ă Ag geometric albedo low 100 parts per million (ppm) on hourly Ag bond albedo Tp pKq planet dayside tempera- timescales [15]. ppm is widely used in as- ture tronomy to display stellar to planetary flux Stellar ´6 ratios that have signals „ 10 . Md mass of sun M˚ pMdq stellar mass Rd radius of sun 2 Theory R˚ pRdq stellar radius F˚ stellar flux Teff pKq effective temperature The transit method is responsible for the Table 1: A list of orbital, planetary and stellar majority of exoplanet discoveries, and with symbols and their names. the expected yield from TESS this propor- tion is expected to rise. The method is particularly insightful with regards to un- covering properties of exoplanets. In ad- dition to an obvious period measurement, dius) from which, coupled with RV plane- the transit depth provides a planetary ra- tary mass measurement, the density can be dius estimate (provided a known stellar ra- computed. Additionally, both the primary (planet occulting star) and secondary (vice versa) transits can provide atmospheric in- formation of the planet. The former giving rise to a detectable absorption spectrum and the latter allowing for a measurement of the planet's true spectrum [19]. The re- curring parameters used throughout are de- Figure 2: The wide bandpass TESS uses, centred on the Ic band. scribed in Table 1. 4 2.1 Transit Fundamentals Shown in Fig. 3 is a schematic of a tran- sit and the resultant light curve. As the planet moves in front of the star periodi- cally, a measurable dip in the light curve of the star is observed (primary transit). Figure 3: A schematic of a transit event and the The secondary transit refers to the planet resultant dip in the light curve (primary transit). orbiting behind the star. Assuming negligi- most exoplanet surveys, this method pref- ble planetary flux, the transit depth is erentially detects low period exoplanets, es- ˆ ˙ 2 Rp pecially in the early stages of a survey. ∆F « : (1) R˚ Typically, ∆F « p1 ´ 2q% for hot Jupiters. Transits are rare, they require an almost edge on (90°) inclination angle (the angle between the observer and the transiting system).
Details
-
File Typepdf
-
Upload Time-
-
Content LanguagesEnglish
-
Upload UserAnonymous/Not logged-in
-
File Pages27 Page
-
File Size-