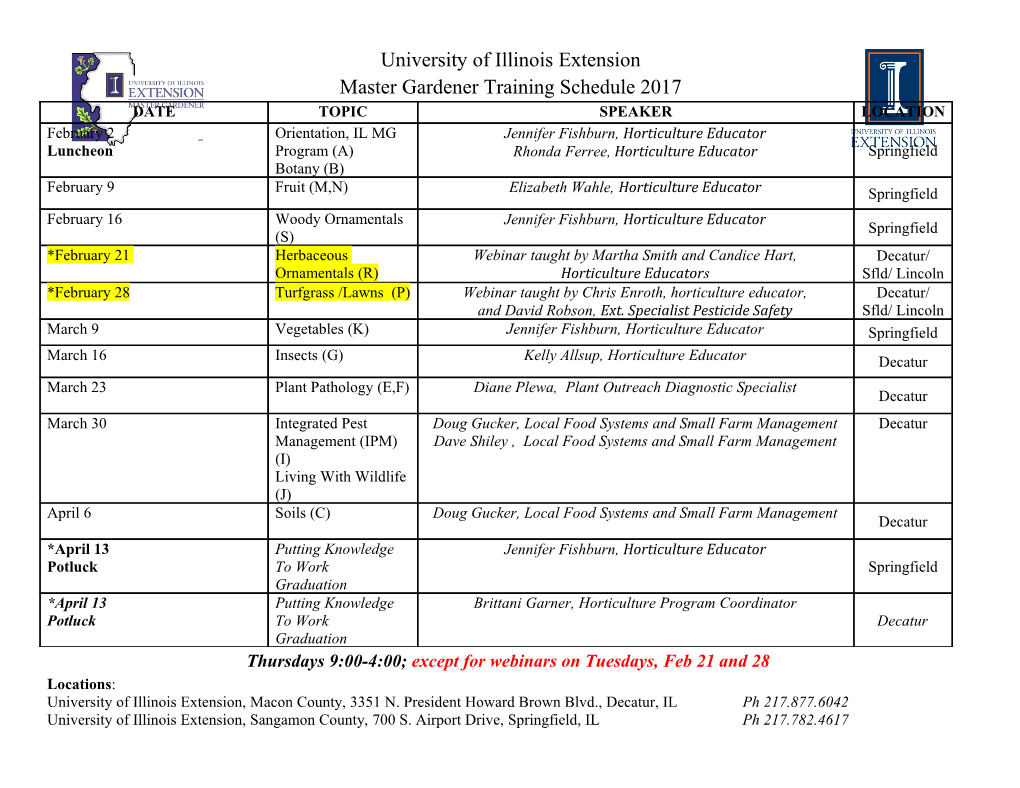
Lecture 1: Options for sustainable development of concrete Professor Karen Scrivener Cement Chemistry for Engineers, Cape Town 31st January 2013 Karen Scrivener • English • 1979 Materials Science, University of Cambridge • 1984 PhD Imperial College, London • 1995 Lafarge, Central Research Laboratory, France • 2001 EPFL, Lausanne, Switzerland • 2002 Creation of Nanocem – Industrial Academic Partnership for Fundamental Research on Cementitious Materials • 2004 Editor in Chief: Cement and Concrete Research 2 Outline The reality about concrete and environmental impact What options do we have Choices for Cement Choices for Concrete 3 Concrete and sustainable development There are a lot of misconceptions about cement and concrete with respect to sustainable development. If we want to improve things we have to start from a correct assessment of the situation We hear a lot about the fact that cement and concrete account for some 5-8% of man-made CO2 What we don’t realise is that this is amazingly good for a material which makes up around half of everything produced. The importance of concrete: Global figures (2005) (a) 34 GT of products (b) 30 GT of CO2e Material Per year A = % of CO2e / yr B = % of a b Concrete 17.1 GT 50.2% 2.6 GT 8.5% Steel* 0.74 GT 2.4% 2.3 GT 8.1% Timber 2.2 GT 6.5% 5.1 GT** 17%** (a) Resource consumption minus: major wastes (agricultural waste, mine tailings); grazed crops; fossil fuels – Krausmann et al Ecol Econ 68 (2009) 2696. (b) Estimate derived from various sources. *Virgin steel not including rebar. **IPCC estimate of emissions owing to forestry operations & thus upper bound. Full details of calculations & data sources available on request. Slide Phil Purnell, University Leeds 5 Concrete is an environmentally friendly material Best able to satisfy the demands of the world population Material MJ/kg kgCO2/kg Cement 4.6 0.83 2 Concrete 0.95 0.13 Masonry 3.0 0.22 Wood 8.5 0.46 Wood: multilayer 15 0.81 Steel: Virgin 35 2.8 Steel: Recycled 9.5 0.43 Aluminium: virgin 218 11.46 Aluminium 28.8 1.69 recycled CO energy, Relative Glass fibre 100 8.1 composites Glass 15.7 0.85 ICE version 1.6a Hammond G.P. and Jones C.I 2008 Proc Instn Civil Engineers www.bath.ac.uk/mech-eng/sert/embodied/ Given these low figures, local supply is key to avoid transport costs 6 Concrete is the only viable solution From these slides we can also see that there is no possible substitute for concrete to satisfy the needs of the worlds population for buildings and infrastructure. For example the amount of wood used per year is about 1/10th the amount of concrete and this level is already judged to be unsustainable – we are cutting down forests faster than we are replanting them. Compressed earth is still used in rural situations, but due to the low strength you need to use much greater quantities than concrete for the same application. Real danger of depleting soil in heavily populated rural areas. Now more the half the people in the world live in urban areas 7 Demand is forecast to rise: to meet the demands of a growing world population more than half of demand is currently in China 8 Concrete and sustainable development So I hope you now believe me that concrete is a very good option from an environment standpoint. But we need to see if we can continue to reduce the environmental footprint AND satisfy the growing demand We need to look at where the associated CO2 emissions are coming from: Origins of CO2 emissions in cement production 1 tonne of cement leads to the emission of 650 – 900 kg CO2 This large range reflects efficiencies of The production process different plants is highly optimised Around 80% of CaCO3 thermodynamic limit. decomposition 40 it is estimated that < 2% (CHEMICAL) further savings can be Fuel made here 60 Use of waste fuels, which can be > 80% reduces the demand CaCO3 CaO + CO2 for fossil fuels NB, importance of being local – sea transport Australia to Europe would add >500 kg /tonne 10 “Chemical CO2” Most of the CO2 emission associated with cement production comes form the decomposition of limestone This situation is particular to cement (and steel) Alternative energy sources will not help, as with most other industrial processes. If we lower this chemical CO2, we will inevitably change the chemistry of the cement And so the way it reacts and performs To introduce low carbon solutions, engineers will have to understand and deal with different cement chemistries. 11 Lets look a bit more at possibilities for low carbon chemistries 12 The resources of the earth mean we do not have a lot of options! S rest Na Mg <2% K rest K Mg Na Ca Fe O Al O ReduceCa Ca Si Si Portland Fe Al earth’s crust cement The composition of the Earth’s Crust limits the possible chemistries But the limited range mean we can explore all options 13 What about the different oxides oxide hydrates geology CaO Good mobility, hydrates can fill space Limestone, widely distributed SiO2 Less soluble but hydrates fill space Most common oxide, everywhere Al2O3 Variable solubility, hydrates fill space With silica in feldspars, clays also / bauxites ? Fe2O3 Low mobility / solubility in alkaline ? Very widely distributed solutions, poor contribution to space filling MgO Low mobility / solubility in alkaline ? More local distribution as major ? solutions, poor contribution to space filling component, wide distribution as minor component Na2O Very soluble, hydrates don’t precipitate Widely distributed as minor - component. K2O Very soluble hydrates don’t precipitate Widely distributed as minor - component. Reaction between water and cement: Increasing solid volume, replaces water Transformation from fluid paste to solid Cement grain hydrates water What about the different oxides oxide hydrates geology CaO Good mobility, hydrates can fill space Limestone,30 year widely old distributed Less soluble but hydrates fill space Most common oxide, everywhere SiO2 concrete Al2O3 Variable solubility, hydrates fill space With silica in feldspars, clays also / bauxites ? Fe2O3 Low mobility / solubility in alkaline ? Very widely distributed solutions, poor contribution to space filling Slag cement MgO Low mobility / solubility in alkaline ? More local distribution as major ? solutions, poor contribution to space filling component, wide distribution as minor component Na2O Very soluble, hydrates don’t precipitate Widely distributed as minor - component. K2O Very soluble hydrates don’t precipitate Widely distributed as minor - component. What about the different oxides oxide hydrates geology CaO Good mobility, hydrates can fill space Limestone, widely distributed SiO2 Less soluble but hydrates fill space Most common oxide, everywhere Al2O3 Variable solubility, hydrates fill space With silica in feldspars, clays also / bauxites ? Fe2O3 Low mobility / solubility in alkaline ? Very widely distributed solutions, poor contribution to space filling MgO Low mobility / solubility in alkaline ? More local distribution as major ? solutions, poor contribution to space filling component, wide distribution as minor component Na2O Very soluble, hydrates don’t precipitate Widely distributed as minor - component. K2O Very soluble hydrates don’t precipitate Widely distributed as minor - component. Most important system CaO-SiO2-Al2O3 SiO2 Silica Fume Natural pozzolan F Fly Ash C Metakaolin Slag Portland Cement Limestone CaO Al2O3 18 Other hydraulic minerals SiO2 C3S 74% CaO (proportional to CO2) C2S 65% CaO CA 35% CaO C4A3$ 37% CaO ? (3CA + C$) - Here we see real potential to reduce CO2 BUT, what sources of minerals are there which contain Al2O3 >> SiO2 ? Bauxite – localised, under Calcium aluminate / increasing demand for Portland calcium sulfo aluminate Aluminium production, Cement EXPENSIVE CaO Al2O3 19 Most promising approach – reducing the clinker factor ↓ CO2 Process optimisation ↓ clinker factor Clinker Gypsum Cement SCMs – Supplementary Cementitious Materials Limestone Fly ash Slag Natural pozzolan Often by-products or wastes from other industries Typical reductions in clinker factor Source: HOLCIM 21 But increasing substitution is reaching a limit due to: - technical performance - uniformity Figures from ~2000 - availability - cost Metakaolin Calcined clays Rice husk ash Silica fume Burnt shale Used in cement Reserve Natural pozzolana Blast furnace slag Fly ash Fly ash: significant volumes with low performance Cement 0 500 1000 1500 2000 2500 Limestone Mill. tons/year 22 So what . Portland cement clinker, blended with SCMs is likely to be best solution for sustainable cements for foreseeable future . Limited supplies of currently widely used SCMs (notably slag and fly ash) . Need solutions adapted to local resources (consequences: broader and more complex products) . Need to find or manufacture new SCMs . Need to better exploit existing materials: . Alumina - limestone synergy . Multiple blends – combining several SCMs . Questions of standards . Issues of variability will increase – need better basic knowledge to handle this 23 EXAMPLE: High substitution possible with combination of metakaolin and limestone Antoni et al, CCR, 2012 • For 45% of substitution, higher mechanical properties from 7 days • For 60% of substitution, mechanical properties still >90% of pure cement • Sulfate optimisation is key, example with B45 shows one day strength can increase by 50%! 24 There is no single magic solution We need to master a diverse range of solutions: optimised according to LOCAL raw materials and applications emissions 2 CO 1990 2000
Details
-
File Typepdf
-
Upload Time-
-
Content LanguagesEnglish
-
Upload UserAnonymous/Not logged-in
-
File Pages33 Page
-
File Size-