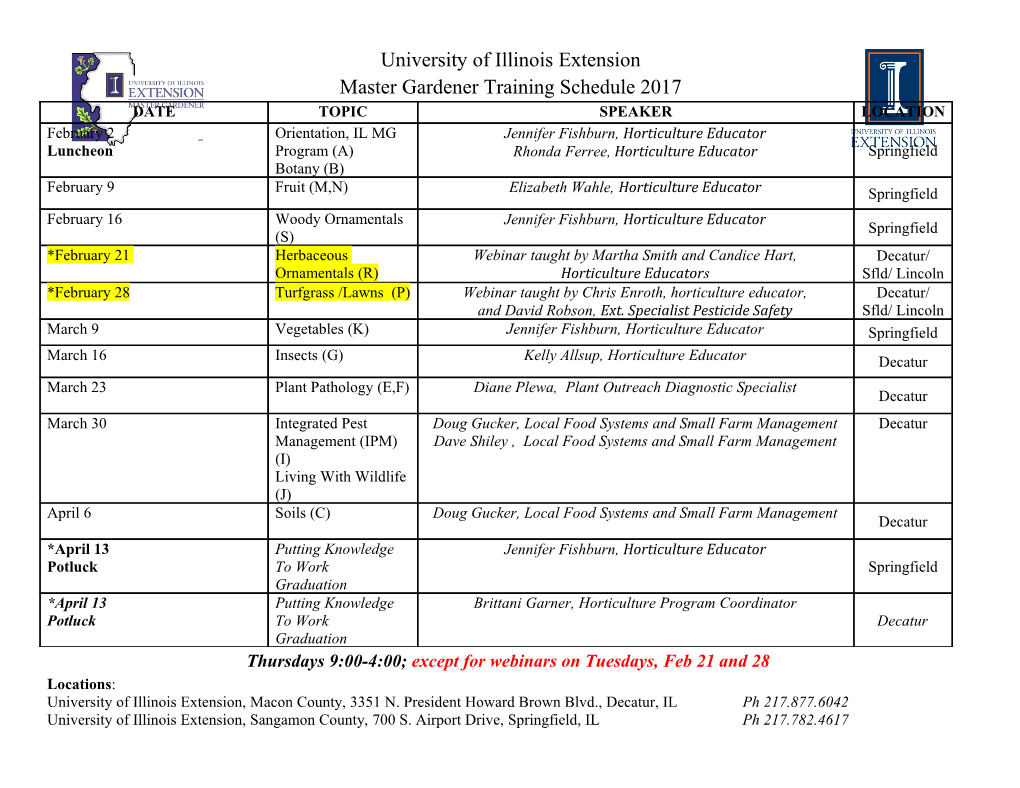
ARTICLE IN PRESS Journal of Insect Physiology 53 (2007) 559–572 www.elsevier.com/locate/jinsphys Modeling cold tolerance in the mountain pine beetle, Dendroctonus ponderosae Jacques Re´ gnie` rea,Ã, Barbara Bentzb aNatural Resources Canada, Canadian Forest Service, Laurentian Forestry Centre, PO Box 10380, Stn. Sainte-Foy, Quebec, QC, Canada G1V 4C7 bUSDA Forest Service, Rocky Mountain Research Station, 860 N 1200 E, Logan, UT 84321, USA Received 11 January 2007; received in revised form 18 February 2007; accepted 19 February 2007 Abstract Cold-induced mortality is a key factor driving mountain pine beetle, Dendroctonus ponderosae, population dynamics. In this species, the supercooling point (SCP) is representative of mortality induced by acute cold exposure. Mountain pine beetle SCP and associated cold-induced mortality fluctuate throughout a generation, with the highest SCPs prior to and following winter. Using observed SCPs of field-collected D. ponderosae larvae throughout the developmental season and associated phloem temperatures, we developed a mechanistic model that describes the SCP distribution of a population as a function of daily changes in the temperature-dependent processes leading to gain and loss of cold tolerance. It is based on the changing proportion of individuals in three states: (1) a non cold- hardened, feeding state, (2) an intermediate state in which insects have ceased feeding, voided their gut content and eliminated as many ice-nucleating agents as possible from the body, and (3) a fully cold-hardened state where insects have accumulated a maximum concentration of cryoprotectants (e.g. glycerol). Shifts in the proportion of individuals in each state occur in response to the driving variables influencing the opposite rates of gain and loss of cold hardening. The level of cold-induced mortality predicted by the model and its relation to extreme winter temperature is in good agreement with a range of field and laboratory observations. Our model predicts that cold tolerance of D. ponderosae varies within a season, among seasons, and among geographic locations depending on local climate. This variability is an emergent property of the model, and has important implications for understanding the insect’s response to seasonal fluctuations in temperature, as well as population response to climate change. Because cold-induced mortality is but one of several major influences of climate on D. ponderosae population dynamics, we suggest that this model be integrated with others simulating the insect’s biology. r 2007 Elsevier Ltd. All rights reserved. Keywords: Cold tolerance; Cold resistance; Cold hardiness; Mountain pine beetle; Winter mortality; Model; Supercooling point 1. Introduction Mortality from cold exposure is one of the key factors in the population dynamics of D. ponderosae,(Yuill, 1941; As a species with a distribution spanning 251 of latitude Reid, 1963; Amman, 1973; Safranyik, 1978; Cole, 1981). and 3000 m of elevation, the mountain pine beetle, Den- Early investigations recognized that adequate acclimation droctonus ponderosae Hopkins (Coleoptera: Curculionidae, to low temperatures could significantly increase this insect’s Scolytinae) occupies a diverse array of habitats, attacking and cold hardiness and survival (Wygant, 1940; Yuill, 1941; reproducing in all Pinus species located throughout the Sømme, 1964). The physiological processes of cold hard- western USA and Canada. Except for a short adult dispersal ening, particularly synthesis of cryoprotectants, are rela- period in summer, the insect’s entire life cycle is spent within tively well understood for insects (see Storey and Storey, the phloem. Although voltinism and synchrony can vary 1988). In many xylophagous insects, cold tolerance changes geographically, larvae are the typical overwintering stage. considerably over the winter (Merivee, 1978; Sømme, 1982), and because of its apparent lack of diapause, the ÃCorresponding author. Tel.: +1 418 648 5257; fax: +1 418 648 5849. cold hardiness of D. ponderosae is probably less dependent E-mail address: [email protected] (J. Re´ gnie` re). on hormonal controls than in many diapausing insects 0022-1910/$ - see front matter r 2007 Elsevier Ltd. All rights reserved. doi:10.1016/j.jinsphys.2007.02.007 ARTICLE IN PRESS 560 J. Re´gnie`re, B. Bentz / Journal of Insect Physiology 53 (2007) 559–572 (Merivee, 1978; Sømme, 1982; Hodkova and Hodek, 1994). substrates can depress an insect’s SCP by 10–20 1C, and In addition, because in this species development occurs accumulation of colligative polyols and antifreeze proteins entirely under the bark, photoperiod is probably not a can depress the SCP by an additional 10 1C(Sømme, 1982; significant cue in the development of cold hardiness. It is Duman et al., 1991). Glycerol is the most abundant likely that the cold-hardening process is controlled mainly antifreeze polyol in D. ponderosae (Sømme, 1964; Bentz by temperature (Sømme, 1982), although Horwath and and Mullins, 1999). Cessation of feeding and voiding the Duman (1986) found that thermoperiod was involved in gut in the fall is a seasonal response that may be mostly antifreeze protein production in Dendroides canadensis as a irreversible in the short term. However, the remaining surrogate to photoperiod. Laboratory evidence (BB unpu- processes such as control of the concentration of intra- and blished data) suggests that daily temperature fluctuations extra-cellular ice-nucleating substances or the synthesis and may be needed to stimulate acclimation to cold in catalysis of antifreeze substances seem to be under D. ponderosae. immediate control of temperature and thus may vary on Many insect species can withstand the formation of ice in a much shorter time scale (Kelleher et al., 1987; Lee et al., the extracellular body fluid and have been labeled freeze 1987; Bale, 2002; Danks, 2005). tolerant (Salt, 1961). Others, including D. ponderosae Using data from early studies on D. ponderosae cold (Bentz and Mullins, 1999) and other scolytine bark beetles tolerance (Wygant, 1940; Yuill, 1941; Sømme, 1964), (Ring, 1977; Gehrken, 1984; Miller and Werner, 1987; Safranyik et al. (1975) developed a model to predict the Lombardero et al., 2000), are freeze intolerant and must probability of outbreaks of this insect in British Columbia. avoid freezing of body tissues. In these species, the In this model, À40 1C is the lethal temperature below which supercooling point (SCP) refers to the temperature at outbreaks come to an abrupt end due to extensive winter which spontaneous nucleation of body water occurs and mortality. Because cold tolerance and cryoprotectant lethal ice crystals form in the insect tissue. The SCP can accumulation are both functions of low-temperature often be 20 1C or more below the freezing point of body acclimation, a more realistic model of survival would fluids, and is often considered a measure of maximum cold describe the dynamic effect of temperature on the insect’s hardiness (Lee, 1989, 1991). In some species, SCP also supercooling capacity. represents the lower lethal temperature (Lombardero et al., While it is clear that modeling the detailed processes of 2000; Tran et al., 2007; Hodkova and Hodek, 1994). It is cold hardening would require far more physiological data widely recognized, however, that pre-freeze mortality at than are currently available for D. ponderosae, our temperatures above the SCP can result in substantial objective was to develop a process-based model of its cold mortality in freeze-avoiding species (Baust and Rojas, tolerance that follows the general physiological principles 1985; Knight et al., 1986; Lee, 1991; Sinclair, 1999; Bale, of cold hardiness outlined above. 2002; Renault et al., 2002). The bark of host trees, in particular lodgepole pine 2. Methods (Pinus contorta), provides little buffer from cold to D. ponderosae living within the phloem (e.g. inner bark). This cold tolerance model was developed from the data Microhabitat temperatures can drop below À35 1C in the set of Bentz and Mullins (1999), consisting of time series of middle of winter and D. ponderosae has no specific hourly phloem temperatures and measurements of the SCP behaviorial traits that allow it to escape from cold. Instead, of individual larvae from six locations sampled throughout it supercools to temperatures well below microhabitat the year in 1992–93 and 1994–95. Individual SCP temperatures (Bentz and Mullins, 1999), suggesting that measurements of 1216 larvae from the four D. ponderosae SCPs are indeed a measure of maximum cold hardiness in instars were available for analysis. One sample (14 January this species. Supercooling capacity fluctuates seasonally 1993, Logan, UT) contained only 2 individuals and was and annually, and is highly variable within and among dropped, leaving 41 samples. The median SCP in each populations (Yuill, 1941; Bentz and Mullins, 1999). While sample (LT50) was determined and one frequency histo- no mortality was observed in larvae exposed for short time gram of the SCP data, pooled over sites, was constructed periods to temperatures slightly above the measured SCP, for each month in which some sampling took place. increased duration at subzero temperatures can cause mortality in D. ponderosae (Safranyik and Linton 1998), 2.1. The model and further investigation into the ecological role of SCPs in D. ponderosae is needed. The monthly distributions of SCPs observed by Bentz and In freeze-avoiding insects, Bale (2002) recognizes two Mullins (1999) (left column, Fig. 1) suggest that distinct processes during cold hardening: (1) ridding the D. ponderosae larvae spend the year in one of three states body of ice-nucleating agents (such as food in the gut and on the basis of their SCP, and that the proportion of the large proteins in the insect’s hemolymph and tissues) and population in each state shifts during the course of the year: (2) accumulating cryoprotectant substances such as poly- hydric alcohols (polyols) and antifreeze proteins (Lee and State 1: summer state, feeding larvae that we refer to as Denlinger, 1991; Duman, 2001). Removal of ice-nucleating non-cold-hardened.
Details
-
File Typepdf
-
Upload Time-
-
Content LanguagesEnglish
-
Upload UserAnonymous/Not logged-in
-
File Pages14 Page
-
File Size-