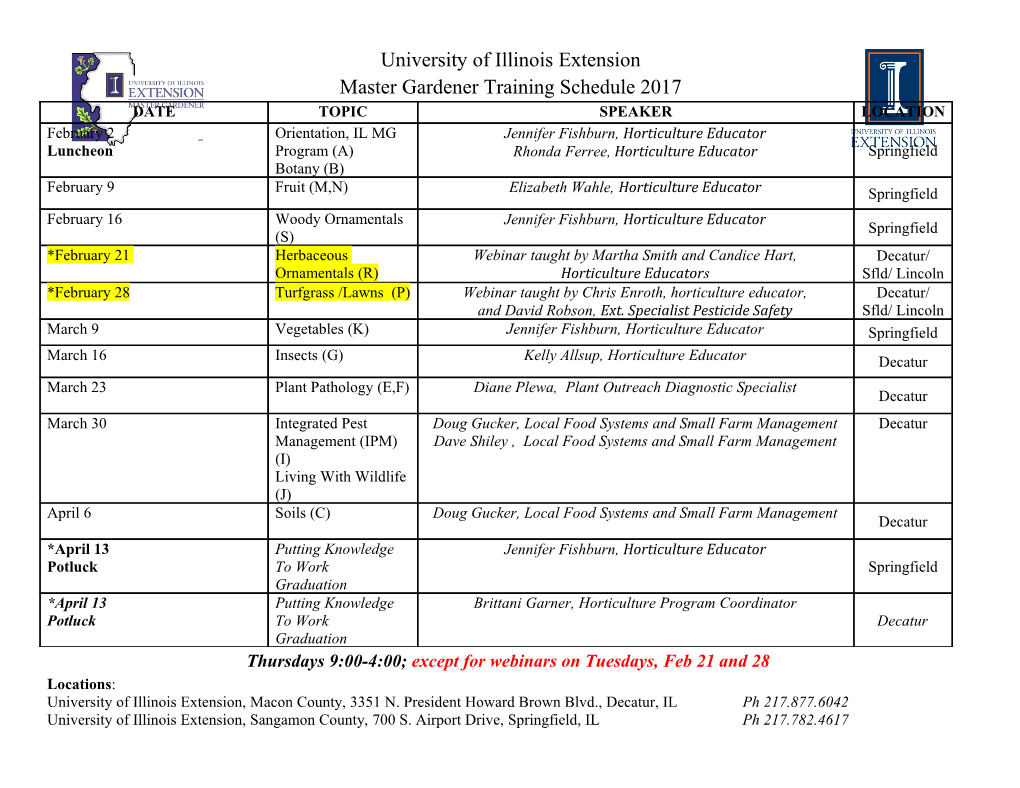
Physics of intraband quantum dot optoelectronic devices Nenad Vukmirovi´c BSc (Hons) The University of Leeds School of Electronic and Electrical Engineering Institute of Microwaves and Photonics June 2007 Submitted in accordance with the requirements for the degree of Doctor of Philosophy. The candidate confirms that the work submitted is his own and that appropriate credit has been given where reference has been made to the work of others. This copy has been supplied on the understanding that it is copyright material and that no quoatation from the thesis may be published without proper acknowledgement. i Acknowledgments I would like to gratefully acknowledge the excellent supervision of Professor Paul Harrison and Dr Dragan Indjin during this work. I also thank Dr Zoran Ikoni´c for sharing his strong experience in physics of nanostructures. Both friendship and scientific support of Dr Ivana Savi´c and Dr Vladimir Jovanovi´c made me feel a member of the group from the first day I arrived at Leeds. I would also like to thank all my office mates for providing a pleasant research atmosphere. The work presented in this thesis would be impossible without the strong theoretical background in physics, mathematics and programming. I am grate- ful to all my teachers from Mathematical High School, Belgrade, as well as Fac- ulty of Physics and Faculty of Electrical Engineering, University of Belgrade. Special thanks to Professor Vitomir Milanovi´c, Faculty of Electrical Engineer- ing, who encouraged me to pursue a PhD in the field of nanostructures at Leeds. Thanks are also due to researchers from other institutions, that I visited or collaborated with. Joint work with colleagues from the Electronic Materials Engineering Department at the Australian National University, Greg Jolley, Satya Barik, Dr Fu Lan and Professor Chennupati Jagadish, during my stay in Canberra in summer 2005, gave me an important insight into the exper- imental procedures for growth and characterisation of realistic quantum dot infrared photodetectors and inspired some of the theoretical works done later. Many useful discussions with Sven H¨ofling, University of Wurzburg,¨ regard- ing the possibility of realisation of quantum dot based cascade structures are also acknowledged. The work presented in Sec. 7.2 was done in collaboration Acknowledgments ii with Pantelis Aivaliotis, Dr Evgeny Zibik and Dr Luke Wilson, University of Sheffield. I would also like to thank Dr Stanko Tomi´c, CCLRC Daresbury Laboratory, for enlightening discussions regarding the methods for quantum dot electronic structure calculations. Thanks also to my friend and colleague Zarkˇ o Gaˇcevi´c for working on his Diploma thesis at the Faculty of Electrical Engineering, University of Belgrade, under my co-supervision, and providing his MATLAB code for comparison. My PhD research was funded by the Overseas Research Students Award Scheme (ORSAS), University of Leeds Tetley and Lupton Scholarship, and the Maintenance grant from the School of Electronic and Electrical Engineering. Additional financial support was obtained from the Institute of Electrical Engi- neers (IEE) in the form of Leslie H Paddle Fellowship and from the Lasers and Electro Optics Society of the Institute of Electrical and Electronics Engineers (IEEE-LEOS) in the form of LEOS Graduate Student Fellowship. Finally, I am indebted to my parents for their understanding and encour- agement when it was most required. iii Abstract In last two decades, semiconductor nanostructures, such as quantum wells, wires and dots, have been recognised as sources and detectors of radiation in the mid- and far-infrared region of the spectrum. Much of a success has been obtained with quantum well based intraband devices, such as quantum cascade lasers and quantum well infrared photodetectors. However due to longer carrier lifetimes in quantum dots, it is expected that optoelectronic devices based on intraband transitions in self-assembled quantum dots would have superior performance to their quantum well counterparts. In order to fully exploit this prospect, appropriate theoretical models describing electronic, optical and transport properties of the active region of these devices need to be developed, which was the subject of this thesis. It was shown how symmetry of the dot shape can be exploited to efficiently calculate the energy levels within the framework of the multiband envelope function method. The implementation of the method in the plane wave rep- resentation of the Hamiltonian eigenvalue problem and the results of its ap- plication to square based pyramidal InAs/GaAs quantum dots and hexagonal III-nitride quantum dots were given. A semiclassical model of intraband carrier dynamics in quantum dots was then developed and applied to design an optically pumped long wavelength mid-infrared laser based on intersublevel transitions in InAs/GaAs quantum dots. Two orders of magnitude lower pumping flux was predicted than in similar quantum well based devices. Next, simulations of the optical absorption spectrum in the existing quan- tum dot infrared photodetector structures were performed. A special emphasis Abstract iv was put into quantum dots-in-a-well structures and explanation of the effect of well width on the detection wavelength. A theory of transport in quantum dot infrared photodetectors starting from the energy levels and wavefunctions obtained by solving the envelope Hamil- tonian, yielding as output the device characteristics such as dark current and responsivity, was then developed. The comparison with experimental data available in the literature was made, yielding a good agreement. Finally, the theory of electron transport through arrays of closely stacked quantum dots, where coherent and polaronic effects become important, there- fore requiring the treatment within the formalism of the nonequilibrium Green's functions, rather than the semiclassical approach, was presented. A design of a structure promising to act as a terahertz quantum dot cascade laser was given. v Contents Acknowledgments i Abstract iii Publications x List of Figures xxi List of Tables xxxi List of Principal Abbreviations xxxii 1 Introduction 1 1.1 Semiconductor quantum dots and their importance . 1 1.2 Optoelectronic devices based on self-assembled quantum dots . 3 1.3 Intraband transitions in quantum nanostructures . 6 1.3.1 Quantum well intersubband devices . 7 1.3.2 Intraband quantum dot devices . 9 1.4 Thesis outline . 12 2 Theoretical framework 14 2.1 Electronic structure of quantum dots . 14 2.2 k p method . 17 · 2.2.1 The 8-band k p Hamiltonian for semiconductors with · zincblende crystal symmetry . 19 Table of Contents vi 2.2.2 The 8-band k p Hamiltonian for semiconductors with · wurtzite crystal symmetry . 25 2.3 The effect of strain . 28 2.3.1 Strain in zincblende crystals . 29 2.3.2 Strain in wurtzite crystals . 31 2.3.3 Piezoelectric effect . 32 2.4 Application of k p Hamiltonian to quantum dot heterostructures 33 · 2.5 The influence of electromagnetic fields . 37 2.5.1 Interaction with external electromagnetic radiation . 37 2.5.2 Spontaneous emission of photons . 39 2.5.3 Static electric and magnetic fields . 41 2.6 Numerical methods . 42 2.6.1 Methods for solving the k p Hamiltonian . 42 · 2.6.2 Methods for finding the strain distribution . 45 2.7 Electron { phonon interaction and polarons . 50 2.7.1 Carrier lifetime in quantum dots . 52 2.7.2 Polaron spectrum in quantum dots . 57 2.8 Nonequilibrium Green's functions theory of transport . 58 3 Electronic structure calculation of square based pyramidal quan- tum dots 63 3.1 Introduction . 63 3.2 The plane wave method . 64 3.3 The symmetry of the model . 66 3.3.1 Representation of the symmetry group operators . 68 3.3.2 The symmetry adapted basis . 71 3.3.3 Piezoelectric effect . 73 3.3.4 Notation of states . 74 3.4 Results . 74 3.4.1 Quantum mechanical coupling and strain distribution . 77 3.4.2 Energy levels in the conduction band . 77 Table of Contents vii 3.4.3 Energy levels in the valence band . 79 3.4.4 Influence of external axial magnetic field . 82 3.5 Conclusion . 84 4 Symmetry based calculation of single-particle states and in- traband absorption in hexagonal GaN/AlN quantum dots 86 4.1 Introduction . 86 4.2 Theoretical framework . 87 4.3 Symmetry considerations . 90 4.4 Results . 94 4.5 Conclusion . 103 5 Proposal of an optically pumped intersublevel laser 105 5.1 Introduction . 105 5.2 Theoretical model . 105 5.3 Results . 111 5.3.1 Active region . 111 5.3.2 Waveguide and cavity design . 117 5.4 Conclusion . 119 6 Absorption spectrum of quantum dot infrared photodetectors121 6.1 Introduction . 121 6.2 Theoretical models of absorption . 122 6.2.1 Parabolic quantum dot model of absorption . 122 6.2.2 Effective mass model of absorption . 124 6.2.3 8-band k p model of absorption . 127 · 6.3 Results . 129 6.4 Conclusion . 137 7 Quantum dots-in-a-well infrared photodetectors 138 7.1 The effect of well width . 138 7.2 Intraband Stark effect in DWELL structures . 144 Table of Contents viii 8 Transport in quantum dot infrared photodetectors 150 8.1 Introduction . 150 8.2 Theoretical model . 151 8.2.1 Modelling the electronic structure . 152 8.2.2 Carrier interaction with phonons and electromagnetic ra- diation . 154 8.2.3 The transport model . 156 8.3 Results . 158 8.3.1 The dark conditions . 159 8.3.2 The light conditions . 163 8.4 Conclusion . 168 9 Nonequilibrium Green's functions theory of transport in quan- tum dot cascades 170 9.1 Introduction . 170 9.2 Theoretical approach . 171 9.3 Validation of the self-consistent Born approximation . 176 9.4 Transport in an ideal superlattice of quantum dots . 181 9.5 The main current peak . 183 9.6 Other resonances .
Details
-
File Typepdf
-
Upload Time-
-
Content LanguagesEnglish
-
Upload UserAnonymous/Not logged-in
-
File Pages269 Page
-
File Size-