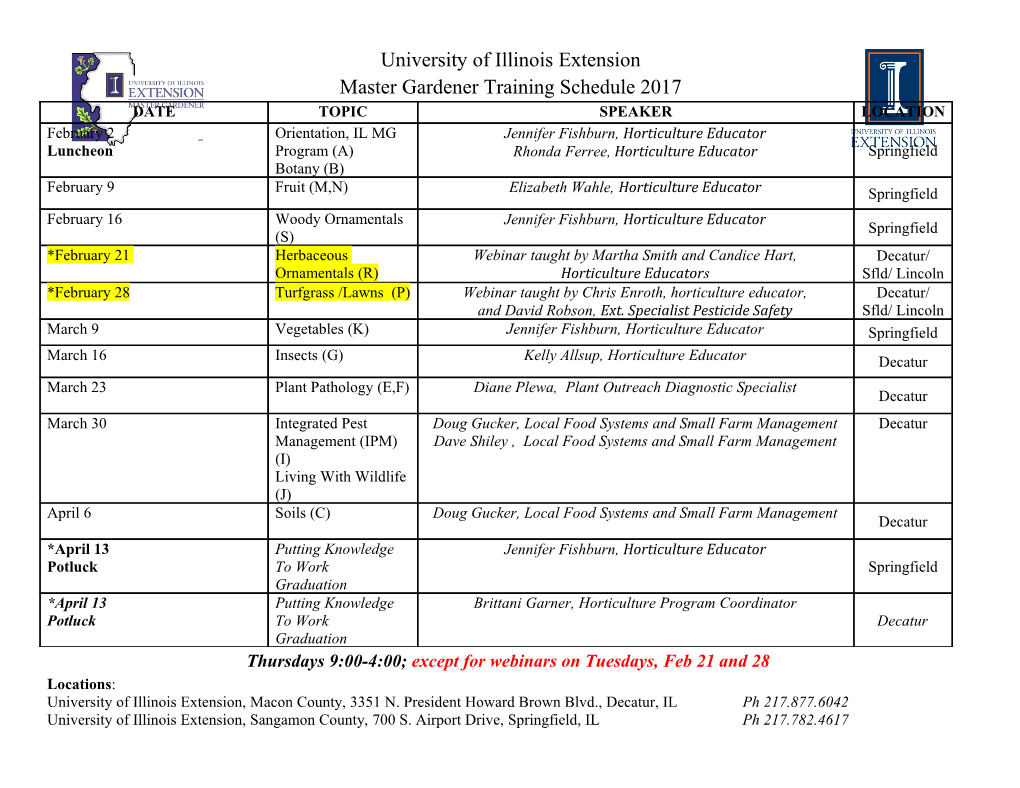
Chapter 5 Menelaus’ theorem 5.1 Menelaus’ theorem Theorem 5.1 (Menelaus). Given a triangle ABC with points X, Y , Z on the side lines BC, CA, AB respectively, the points X, Y , Z are collinear if and only if BX CY AZ = 1. XC · YA · ZB − A Y Z W X B C Proof. (= ) Let W be the point on AC such that BW//XY . Then, ⇒ BX WY AZ AY = , and = . XC YC ZB YW It follows that BX CY AZ WY CY AY CY AY WY = = = 1. XC · YA · ZB YC · YA · YW YC · YA · YW − ( =) Suppose the line joining X and Z intersects AC at Y ′. From above, ⇐ BX CY AZ BX CY AZ ′ = 1= . XC · Y ′A · ZB − XC · YA · ZB It follows that CY CY ′ = . Y ′A YA The points Y ′ and Y divide the segment CA in the same ratio. These must be the same point, and X, Y , Z are collinear. 202 Menelaus’ theorem Example 5.1. The external angle bisectors of a triangle intersect their opposite sides at three collinear points. Y ′ A c b C X′ B a Z′ Proof. If the external bisectors are AX′, BY ′, CZ′ with X′, Y ′, Z′ on BC, CA, AB respectively, then BX c CY a AZ b ′ = , ′ = , ′ = . X′C −b Y ′A − c Z′B −a BX′ CY ′ AZ′ It follows that = 1 and the points X′, Y ′, Z′ are collinear. X′C · Y ′A · Z′B − 5.2 Centers of similitude of two circles 203 5.2 Centers of similitude of two circles Given two circles O(R) and I(r), whose centers O and I are at a distance d apart, we animate a point X on O(R) and construct a ray through I oppositely parallel to the ray OX to intersect the circle I(r) at a point Y . The line joining X and Y intersects the line OI of centers at a point T which satisfies OT : IT = OX : IY = R : r. This point T is independent of the choice of X. It is called the internal center of similitude, or simply the insimilicenter, of the two circles. Y T T ′ O I Y X ′ If, on the other hand, we construct a ray through I directly parallel to the ray OX to intersect the circle I(r) at Y ′, the line XY ′ always intersects OI at another point T ′. This is the external center of similitude, or simply the exsimilicenter, of the two circles. It divides the segment OI in the ratio OT ′ : T ′I = R : r. − 5.2.1 Desargue’s theorem Given three circles with centers A, B, C and distinct radii, show that the exsimilicenters of the three pairs of circles are collinear. A B Z C Y X 204 Menelaus’ theorem 5.3 Ceva’s theorem Theorem 5.2 (Ceva). Given a triangle ABC with points X, Y , Z on the side lines BC, CA, AB respectively, the lines AX, BY , CZ are concurrent if and only if BX CY AZ = +1. XC · YA · ZB A Z Y P B X C Proof. (= ) Suppose the lines AX, BY , CZ intersect at a point P . Consider the line BPY cutting⇒ the sides of triangle CAX. By Menelaus’ theorem, CY AP XB CY PA BX = 1, or = +1. YA · PX · BC − YA · XP · BC Also, consider the line CPZ cutting the sides of triangle ABX. By Menelaus’ theorem again, AZ BC XP AZ BC XP = 1, or = +1. ZB · CX · PA − ZB · XC · PA Multiplying the two equations together, we have CY AZ BX = +1. YA · ZB · XC ( =) Exercise. ⇐ 5.4 Some triangle centers 205 5.4 Some triangle centers 5.4.1 The centroid If D, E, F are the midpoints of the sides BC, CA, AB of triangle ABC, then clearly AF BD CE =1. FB · DC · EA The medians AD, BE, CF are therefore concurrent. Their intersection is the centroid G of the triangle. Consider triangle ADC with transversal BGE. By Menelaus’ theorem, AG DB CE AG 1 1 1= = − . − GD · BC · EA GD · 2 · 1 It follows that AG : GD =2:1. The centroid of a triangle divides each median in the ratio 2:1. A A Y F E Z G I B D C B X C 5.4.2 The incenter Let X, Y , Z be points on BC, CA, AB such that AX, BY , CZ bisect angles BAC, CBA and ACB respectively. Then AZ b BX c CY a = , = , = . ZB a XC b YA c It follows that AZ BX CY b c a = = +1, ZB · XC · YA a · b · c and AX, BY , CZ are concurrent. Their intersection is the incenter of the triangle. Applying Menelaus’ theorem to triangle ABX with transversal CIZ, we have AI XC BZ AI b a AI b + c 1= = − = = . − IX · CB · ZA IX · b + c · b ⇒ IX a 206 Menelaus’ theorem 5.4.3 The Gergonne point Let the incircle of triangle ABC be tangent to the sides BC at X, CA at Y , and AB at Z respectively. Since AY = AZ = s a, BZ = BX = s b, and CX = CY = s c, we have − − − BX CY AZ s b s c s a = − − − =1. XC · YA · ZB s c · s a · s b − − − By Ceva’s theorem, the lines AX, BY , CZ are concurrent. The intersection is called the Gergonne point Ge of the triangle. A s a − s a − Y Z I s c Ge − s b − B s b X s c C − − Lemma 5.3. The Gergonne point Ge divides the cevian AX in the ratio AG a(s a) e = − . G X (s b)(s c) e − − Proof. Applying Menelaus’ theorem to triangle ABX with transversal CGeZ, we have AG XC BZ AG (s c) s b AG a(s a) 1= e = e − − − = e = − . − G X · CB · ZA G X · a · s a ⇒ G X (s b)(s c) e e − e − − 5.4 Some triangle centers 207 5.4.4 The Nagel point If X′, Y ′, Z′ are the points of tangency of the excircles with the respective sidelines, the lines AX′, BY ′, CZ′ are concurrent by Ceva’s theorem: BX′ CY ′ AZ′ s c s c s a = − − − =1. X C · Y A · Z B s b · s a · s b ′ ′ ′ − − − The point of concurrency is the Nagel point Na. Ib A s b s c Ic − − Z′ Y ′ Na s a s a − − B s c X s b C − ′ − Ia Lemma 5.4. If the A-excircle of triangle ABC touches BC at X′, then the Nagel point divides the cevian AX′ in the ratio AN a a = . N X s a a ′ − Proof. Applying Menelaus’ theorem to triangle ACX′ with transversal BNaY ′, we have AN X′B CY ′ AN (s c) s a AN a 1= a = a − − − = a = . − N X · BC · Y A N X · a · s c ⇒ N X s a a ′ ′ a ′ − a ′ − 208 Menelaus’ theorem 5.5 Isotomic conjugates Given points X on BC, Y on CA, and Z on AB, we consider their reflections in the midpoints of the respective sides. These are the points X′ on BC, Y ′ on CA and Z′ on AB satisfying BX′ = XC, BX = X′C; CY ′ = Y A, CY = Y ′A; AZ′ = ZB, AZ = Z′B. Clearly, AX, BY , CZ are concurrent if and only if AX′, BY ′, CZ′ are concurrent. A Z ′ Y Y Z ′ P P • B C X X′ Proof. BX CY AZ BX CY AZ BX BX CY CY AZ AZ ′ ′ ′ = ′ ′ ′ = 1. XC · Y A · ZB X C · Y A · Z B XC · X C Y A · Y A ZB · Z B ′ ′ ′ ′ ′ ′ The points of concurrency of the two triads of lines are called isotomic conjugates. 5.5 Isotomic conjugates 209 Example 5.2. (The Gergonne and Nagel points) Ib A Ic Z′ Y Z I Y ′ Na Ge B C X X′ Ia Example 5.3. (The isotomic conjugate of the orthocenter) Let H• denote the isotomic conjugate of the orthocenter H. Its traces are the pedals of the reflection of H in O. This latter point is the deLongchamps point Lo. A Z′ Y Lo O Y ′ H• Z H B C X X′ 210 Menelaus’ theorem Example 5.4. (Yff-Brocard points) Consider a point P =(u : v : w) with traces X, Y , Z satisfying BX = CY = AZ = µ. This means that w u v a = b = c = µ. v + w w + u u + v Elimination of u, v, w leads to 0 µ a µ − − 0= b µ 0 µ =(a µ)(b µ)(c µ) µ3. − − − − − − µ c µ 0 − − Indeed, µ is the unique positive root of the cubic polynomial (a t)(b t)(c t) t3. − − − − This gives the point 1 1 1 c µ 3 a µ 3 b µ 3 P = − : − : − . b µ c µ a µ − − − ! A A Z′ Y ′ Z Y P • P B C B C X X′ The isotomic conjugate 1 1 1 b µ 3 c µ 3 a µ 3 P • = − : − : − c µ a µ b µ − − − ! has traces X′, Y ′, Z′ that satisfy CX′ = AY ′ = BZ′ = µ. These points are called the Yff-Brocard points. 1 They were briefly considered by A. L. Crelle. 2 1P. Yff, An analogue of the Brocard points, Amer. Math. Monthly, 70 (1963) 495 – 501. 2A. L. Crelle, 1815..
Details
-
File Typepdf
-
Upload Time-
-
Content LanguagesEnglish
-
Upload UserAnonymous/Not logged-in
-
File Pages10 Page
-
File Size-