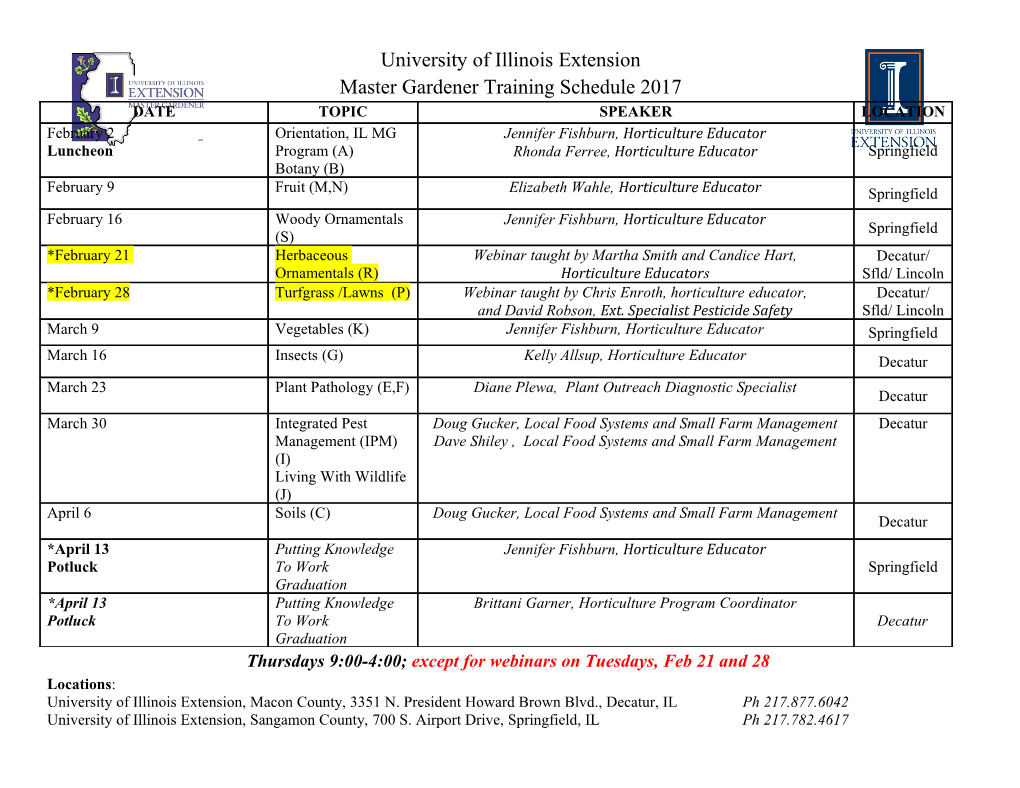
View metadata, citation and similar papers at core.ac.uk brought to you by CORE provided by Elsevier - Publisher Connector Discrete Optimization 2 (2005) 145–153 www.elsevier.com/locate/disopt Partitioning cographs into cliques and stable sets Marc Demangea, Tınaz Ekimb,∗,1, Dominique de Werrab aESSEC, Département SID, France bEPFL, Recherche Opérationnelle Sud Est (ROSE), 1015Lausanne, Switzerland Received 10 May 2004; received in revised form 30 November 2004; accepted 8 March2005 Available online 5 July 2005 Abstract We consider the problem of partitioning the node set of a graph into p cliques and k stable sets, namely the (p, k)-coloring problem. Results have been obtained for general graphs [Feder et al., SIAM J. Discrete Math. 16 (3) (2003) 449–478], chordal graphs [Hell et al., Discrete Appl. Math. 141 (2004) 185–194] and cacti for the case where k = p in [Ekim and de Werra, On split-coloring problems, submitted for publication] where some upper and lower bounds on the optimal value minimizing k are also presented. We focus on cographs and devise some efficient algorithms for solving (p, k)-coloring problems and cocoloring / problems in O(n2 + nm) time and O(n3 2) time, respectively. We also give an algorithm for finding the maximum induced (p, k)-colorable subgraph. In addition to this, we present characterizations of (2, 1)- and (2, 2)-colorable cographs by forbidden configurations. © 2005 Elsevier B.V. All rights reserved. Keywords: (p, k)-coloring; Cocoloring; Split-coloring; Cographs 1. Introduction Partitioning the node set of a graph into two kinds of subsets (like for instance cliques and stable sets) is a natural extension of the classical coloring problem which has therefore been a topic of extensive research in the recent years (see [1,2,5,6,10,11]). Choosing subsets of nodes that induce cliques and stable sets has in addition the nice property of complementarity: the problem is basically the same whether we consider the graph G or its complement. In a more general context, Feder et al. [6] have considered so-called dense graphs and sparse graphs (instead of collection of cliques and collection of stable sets) and they have studied the problem of partitioning the node set of a graph G into a dense graphand a sparse graph.Complexity results are also given in [6]. Along the same line, Hell et al. [10] consider the problem of partitioning the node set into p cliques and k stable sets and examine in particular the case of chordal graphs. These are the graphs in which every cycle of length at least 4 contains a chord (an edge linking two nonadjacent nodes of a cycle). They devise polynomial time algorithms using a perfect elimination order defined on a chordal graph for solving the above mentioned partitioning problems. As a special case when k = p, the problem consists in partitioning the node set into k split graphs (these are graphs in which the node set is the union of a clique and a stable set). It has been studied in [5]. ∗ Corresponding author. Tel.: +41 21 693 25 68; fax: +41 21 693 58 40. E-mail address: tinaz.ekim@epfl.ch (T. Ekim). 1 The research of Tınaz Ekim was supported by Grant 200021-101455/1 of the Swiss National Science Foundation whose support is greatly acknowledged. 1572-5286/$ - see front matter © 2005 Elsevier B.V. All rights reserved. doi:10.1016/j.disopt.2005.03.003 146 M. Demange et al. / Discrete Optimization 2 (2005) 145–153 In this paper we intend to discuss the problem of partitioning the node set of a graph into p cliques and k stable sets; we call (p, k)-coloring problems all different versions of this partitioning problem. We shall first review some basic results concerning suchpartitioning in general graphs [6] and after that we shall derive and exploit some consequences. We will consider cographs also called P4-free graphs since they are defined as graphs without induced chain on four nodes, i.e., without P4. These are special cases of permutation graphs (graphs G for which G and its complement have a transitive orientation). We shall give a new characterization of cographs which lead to efficient algorithms for all (p, k)-coloring problems. General remarks on these (p, k)-coloring problems will also be given as well as some avenues for further research. Given a graph G = (V, E), |V |=n and |E|=m. We denote by G the complement of G.ForV ⊂ V , G[V ] denotes the subgraphof G induced by V . A graph G=(V, E) is (p, k)-colorable if V can be partitioned into p cliques and k stable sets of G: p k i j V = (K1,...,K ,S1,...,S ) where K , i = 1,...,pis a clique and S , j = 1,...,kis a stable set. Sucha partition is called a (p, k)-coloring of G.Ifp = k, then it is called a k-split-coloring of G [5]. Another problem, called cocoloring [9,7], consists in minimizing the total number of cliques and/or stable sets covering all the nodes of a graph. In more general (p, k)-coloring problems, we minimize k (resp. p)forafixedp (resp. k). We denote by KpSk the class of (p, k)-colorable graphs. Finally, given a class C of graphs, C ∩ KpSk is said to be polynomially determined if there exists a polynomial-time algorithm computing, for every graph G ∈ C,a(p, k)-coloring of G whenever G ∈ KpSk or recognizing that G/∈ KpSk. Given a graph G, we denote by p,k(G) the maximum cardinality (number of nodes) of a (p, k)-colorable subgraphof G.By convention, 0,0(G) = 0. Note that 1,1(G) is called the split-independence number of G and denoted by S(G) in [5]. A class G of graphs is called hereditary if every node-induced subgraphof a graph G ∈ G also belongs to G. 2. (p, k)-coloring general graphs In this section, we focus on (p, k)-colorings of general graphs. K0S1 (stable sets) and K1S0 (cliques) are trivially poly- nomially determined as well as K0S2 (bipartite graphs) and K2S0 (co-bipartite graphs). Let us also note that a graph G is (p, k)-colorable if and only if its complement G is (k, p)-colorable; consequently for every class of graphs C closed under complementation, KpSk ∩ C is polynomially determined if and only if KkSp ∩ C is polynomially determined. The following theorem is proved in [6]: Theorem 1 (Feder et al. [6]). Let G be a hereditary class of graphs, let (p, k) ∈ N×N, k 1. If K0Sk ∩G and KpS0 ∩G are polynomially determined, then KpSk ∩G is polynomially determined. Moreover, if KpS0 ∩G (resp. K0Sk ∩G) is determined kp+ in time Tp(n) (resp. Tk(n)), then KpSk ∩ G can be determined in time O((n2 2) max(Tp(n), Tk(n))). Corollary 2. If G is a subclass of perfect graphs, then KpSk ∩ G is polynomially determined. This follows also from the fact that hereditary families of perfect (p, k)-colorable graphs have a finite forbidden subgraph characterization (and hence a polynomial time recognition algorithm) [11]. For instance, KpSk ∩ G can be determined in the kp+ class of cographs in time O(mn2 2), where m denotes the number of edges and n the number of nodes. A special case of Theorem 1 is the test of (i, j)-colorability with i, j 2; note that the cases (0, 2) and (2, 0) reduce to testing for the existence of a node 2-coloring. Corollary 3 (Brandstädt [1] and Brandstädt et al. [2]). Since KiS0 and K0Sj are polynomially determined for i, j 2, K2S2 is also polynomially determined. Let us note that the condition “G is hereditary” in Theorem 1 is needed unless NP = P. In fact, let us consider the class G of graphs obtained by adding to any graph G a 4-clique K4 (a clique of size 4) without any edge between G and this clique. G∩K0S3 is trivially polynomially determined since suchgraphsare never (0, 3)-colorable. On the other hand, G∩K1S0 is also polynomially determined (recognition of a clique). But it is NP-complete to decide whether a graph of G is (1, 3)-colorable. In fact a graph G is (0, 3)-colorable if and only if the graph obtained by adding to G a 4-clique not connected to G is (1, 3)-colorable. Let us also point out that recognizing a (1, 3)-coloring remains difficult even if a maximum induced (p, k)-colorable subgraph with (p, k) equal to (1, 0), (0, 1), (0, 2) and (0, 3) can be polynomially computed: Proposition 4. There exists a class of graphs G such that a maximum clique, a maximum stable set, a maximum induced bipartite and also a maximum 3-colorable induced subgraph can be polynomially computed on G while it is NP-complete to decide whether a graph in G is (1, 3)-colorable. M. Demange et al. / Discrete Optimization 2 (2005) 145–153 147 Proof. Let us revisit the proof of NP-completeness of graph 3-colorability by reduction to 3-SAT [8]. Given an instance of 3-SAT with p clauses (C1,...,Cp) and n boolean variables (x1,...,xn), one constructs a graph G = (V, E) defined by V = V1 ∪ V2 ∪ V3, V ={R,T,F} V = n {x , x¯ } V = p {yj ,yj ,yj ,yj ,yj } where 1 , 2 i=1 i i and 3 j=1 1 2 3 4 5 . V contains two nodes per literal, five nodes per clause and three color nodes R,T,F (standing for Red, True and False).
Details
-
File Typepdf
-
Upload Time-
-
Content LanguagesEnglish
-
Upload UserAnonymous/Not logged-in
-
File Pages9 Page
-
File Size-