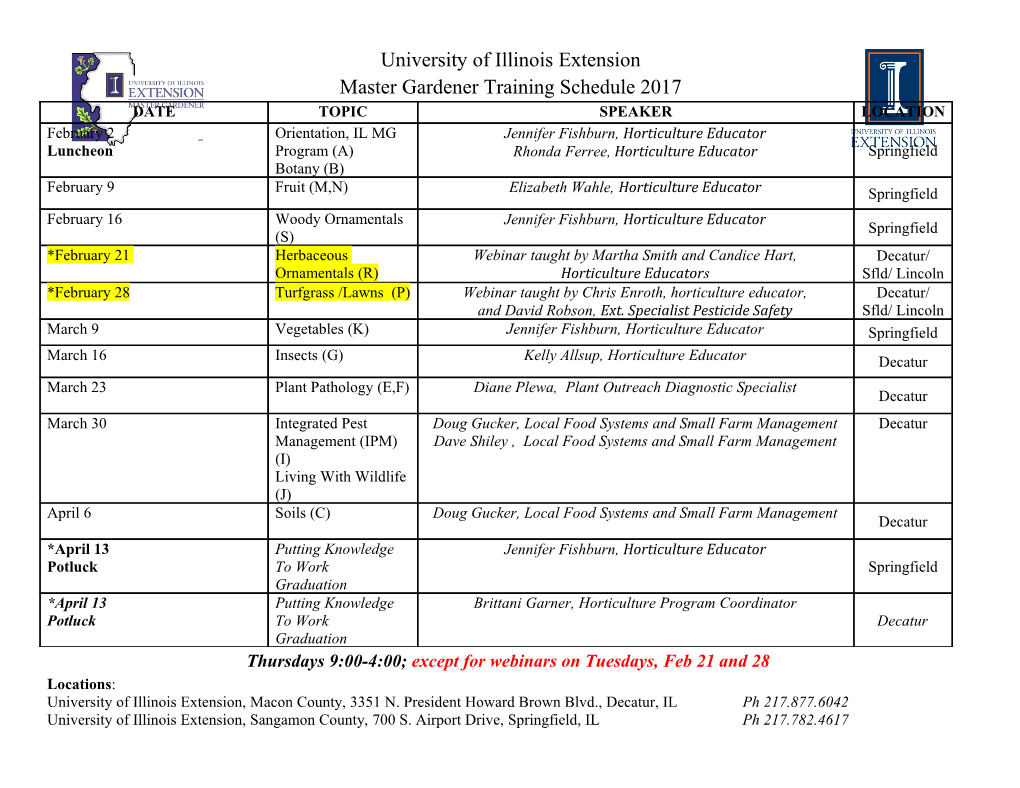
The Cryosphere, 15, 3637–3654, 2021 https://doi.org/10.5194/tc-15-3637-2021 © Author(s) 2021. This work is distributed under the Creative Commons Attribution 4.0 License. The 21st-century fate of the Mocho-Choshuenco ice cap in southern Chile Matthias Scheiter1,a, Marius Schaefer2, Eduardo Flández3, Deniz Bozkurt4,5, and Ralf Greve6,7 1Research School of Earth Sciences, Australian National University, Canberra, Australia 2Instituto de Ciencias Físicas y Matemáticas, Universidad Austral de Chile, Valdivia, Chile 3Departamento de Física, Facultad de Ciencias, Universidad de Chile, Santiago, Chile 4Departamento de Meteorología, Universidad de Valparaíso, Valparaíso, Chile 5Center for Climate and Resilience Research (CR)2, Santiago, Chile 6Institute of Low Temperature Science, Hokkaido University, Sapporo, Japan 7Arctic Research Center, Hokkaido University, Sapporo, Japan aformerly at: Institut für Geophysik und Geoinformatik, TU Bergakademie Freiberg, Freiberg, Germany Correspondence: Matthias Scheiter ([email protected]) Received: 8 October 2020 – Discussion started: 11 November 2020 Revised: 23 June 2021 – Accepted: 25 June 2021 – Published: 6 August 2021 Abstract. Glaciers and ice caps are thinning and retreat- different global climate models and on the uncertainty asso- ing along the entire Andes ridge, and drivers of this mass ciated with the variation of the equilibrium line altitude with loss vary between the different climate zones. The south- temperature change. Considering our results, we project a ern part of the Andes (Wet Andes) has the highest abun- considerable deglaciation of the Chilean Lake District by the dance of glaciers in number and size, and a proper under- end of the 21st century. standing of ice dynamics is important to assess their evo- lution. In this contribution, we apply the ice-sheet model SICOPOLIS (SImulation COde for POLythermal Ice Sheets) to the Mocho-Choshuenco ice cap in the Chilean Lake Dis- 1 Introduction trict (40◦ S, 72◦ W; Wet Andes) to reproduce its current state and to project its evolution until the end of the 21st cen- Most glaciers and ice caps in the Andes are currently thin- tury under different global warming scenarios. First, we cre- ning and retreating (e.g. Braun et al., 2019), and rates of mass ate a model spin-up using observed surface mass balance loss are increasing in many places (Dussaillant et al., 2019). ◦ data on the south-eastern catchment, extrapolating them to In the southernmost part of the Andes (36–56 S), which is the whole ice cap using an aspect-dependent parameteriza- called the Wet Andes or Patagonian Andes in the literature tion. This spin-up is able to reproduce the most important (Lliboutry, 1998), the highest number of glaciers are found, present-day glacier features. Based on the spin-up, we then and large ice fields such as the Northern Patagonia Ice Field, run the model 80 years into the future, forced by projected Southern Patagonia Ice Field, and Cordillera Darwin are lo- surface temperature anomalies from different global climate cated in this region. The specific mass losses observed or in- models under different radiative pathway scenarios to obtain ferred for the glaciers of the Wet Andes are the highest in estimates of the ice cap’s state by the end of the 21st cen- the Andes (Dussaillant et al., 2019; Braun et al., 2019) and tury. The mean projected ice volume losses are 56 ± 16 % among the highest of all glacier regions worldwide (Zemp (RCP2.6), 81 ± 6 % (RCP4.5), and 97 ± 2 % (RCP8.5) with et al., 2019). respect to the ice volume estimated by radio-echo sounding The maritime climate of the Wet Andes is characterized − data from 2013. We estimate the uncertainty of our projec- by high precipitation rates of up to 10myr 1 on the wind- tions based on the spread of the results when forcing with ward side and rather mild temperatures with freezing levels generally above 1 km above mean sea level with an over- Published by Copernicus Publications on behalf of the European Geosciences Union. 3638 M. Scheiter et al.: The 21st-century fate of the Mocho-Choshuenco ice cap in southern Chile all modest seasonality (Garreaud et al., 2013). This leads Table 1. Comparison between simulated and observed velocities at to an exceptionally high mass turnover (Schaefer et al., stakes where velocity observations are available from Geoestudios 2013, 2015, 2017) and high flow speeds for the glaciers in the (2013). region (Sakakibara and Sugiyama, 2014; Mouginot and Rig- not, 2015). In addition to climate forcings, other important Stake B8 B10 B12 B14 B15 B17 B18 −1 contributors to glacier change in the region are ice dynamics vobs (myr ) 22.2 12.7 60.3 33.8 19.4 31.2 27.2 −1 and frontal ablation. Ice-flow models incorporate these pro- vsim (myr ) 11.6 13.7 35.9 20.2 20.7 18.6 20.5 cesses and are therefore appropriate tools to project the future behaviour of the glaciers of the Wet Andes. Only a few studies have tried to project future be- We conclude the paper by summarizing the main findings in haviour of Andean glaciers. Réveillet et al.(2015) mod- Sect. 5. elled Zongo Glacier (16◦ S) in the tropical Andes using the three-dimensional full-Stokes model Elmer/Ice (developed by Gagliardini et al., 2013). They projected volume losses 2 Methods between 40 % and 89 % by the end of this century under the RCP2.6 and RCP8.5 scenarios, respectively. In the Wet 2.1 Observational data Andes, Möller and Schneider(2010) projected an area loss The ice cap on which we focus in this study covers the of 35 % of Glaciar Noroeste, an outlet glacier of the Gran Mocho-Choshuenco volcanic complex, which is located at Campo Nevado ice cap (53◦ S), by the end of the 21st century 40◦ S, 72◦ W (see inset map in Fig.1). Over the last using a degree-day model and volume-area scaling relation- 20 years, climatological and glaciological observations have ships. Schaefer et al. (2013) modelled the surface mass bal- been made on the ice cap (Rivera et al., 2005; Schaefer ance (SMB) of the Northern Patagonian Ice Field in the 21st et al., 2017). SMB data were obtained through the traditional century under the A1B scenario (of Assessment Report 4 glaciological method on a stake network on the south-eastern from the Intergovernmental Panel on Climate Change, IPCC; part of the ice cap (red stars in Fig.1). These measurements comparable to RCP6.0). They projected a strongly decreas- reported by Schaefer et al.(2017) yielded an average neg- ing SMB until the end of the 21st century mainly due to an ative SMB of −0:9mw:e:yr−1 (metre water equivalent per increase in surface temperature by the middle of the century year) with a high mass turnover of around 2:6mw:e:yr−1 and a decrease in accumulation towards the end of the cen- (see Sect. 2.4). This high mass turnover is a consequence tury. Collao-Barrios et al.(2018) infer important committed of the interaction between high precipitation rates leading mass loss of San Rafael Glacier under current climate apply- to high accumulation rates and high temperatures leading to ing the Elmer/Ice flow model with fixed glacier outlines. high melt rates. In this respect, climatological data (2006 to In this contribution, our first objective is to reproduce the 2015) indicate that the annual mean temperature was 2:6 ◦C present-day behaviour of the Mocho-Choshuenco ice cap in at an automatic weather station (green circle in Fig.1) at an the northern part of the Wet Andes (40◦ S) using the ice- elevation of 2000m and therefore close to the typical equilib- sheet model SICOPOLIS (SImulation COde for POLyther- rium line altitude (ELA) (Schaefer et al., 2017). Mean annual mal Ice Sheets) (Greve, 1997a,b). To this end, we make precipitation over the same period was around 4000mmyr−1 use of a newly developed SMB parameterization scheme and in Puerto Fuy at an elevation of 600m to the north of the vol- glaciological data obtained on the ice cap to calibrate the cano, and orographic precipitation effects lead to a relatively model and reproduce its current state. Our second objective high amount of precipitation on the ice cap. is to project the behaviour of the Mocho-Choshuenco ice cap At some of the mass balance stakes (red stars with inner through the course of the 21st century to provide one of the black dots in Fig.1), high precision GPS measurements were first constraints on future glacier dynamics in the Wet Andes. made in July and October 2013 to infer surface flow velocity For this aim, we make use of temperature projections from (Geoestudios, 2013), and the observed velocities are shown 23 global climate models (GCMs) participating in the Cou- in Table1. Further measurements include ground penetrating pled Model Intercomparison Project phase 5 (CMIP5) (Tay- radar (GPR) transects (green lines in Fig.2a) over most parts lor et al., 2012) under low (RCP2.6), medium (RCP4.5), and of the ice cap (Geoestudios, 2014). Through inverse distance high (RCP8.5) emission scenarios as input to SICOPOLIS. weighting interpolation over the whole ice cap, a total ice We begin this paper by describing the observational data volume of 1:038km3 was obtained (Geoestudios, 2014). The and methods (Sect. 2). In Sect. 3, we present the results. First, interpolated ice thickness map was subtracted from a dig- we validate the model spin-up using observed SMB, glacier ital elevation model (TanDEM WorldDEM™, acquired be- outlines, ice thickness, and flow speed. We then present the tween 2012 and 2014) to yield a bedrock topography (Flán- evolution of ice cap extension and volume during the 21st dez, 2017).
Details
-
File Typepdf
-
Upload Time-
-
Content LanguagesEnglish
-
Upload UserAnonymous/Not logged-in
-
File Pages18 Page
-
File Size-