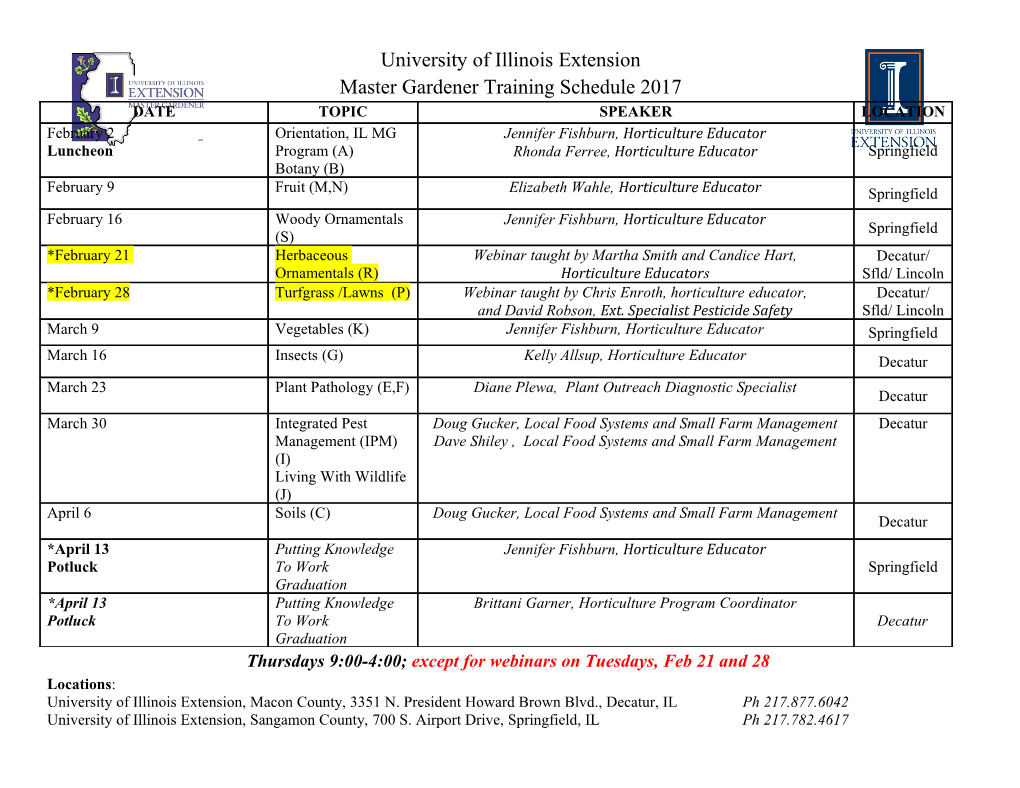
Test of an ALFA prototype detector at the CERN SPS MASTERARBEIT zur Erlangung des akademischen Grades Master of Science (M.Sc. Phys.)im Fach Physik eingereicht an der Mathematisch-Naturwissenschaftlichen Fakultät I Institut für Physik Humboldt-Universität zu Berlin von Yohany Rodríguez García geboren am 14.09.1973 in Bogotá - Kolumbien Präsident der Humboldt-Universität zu Berlin: Prof. Dr. Jan-Hendrik Olbertz Gutachter: 1. Herr Prof. Dr. Thomas Lohse 2. Herr Dr. Ulrich Husemann eingereicht am: 05. April 2011 Abstract ALFA (Absolute Luminosity for ATLAS) is a high-precision scintillating fibre de- tector which has been designed to determine the LHC absolute luminosity at the ATLAS interaction point. This detector consists of eight Roman Pots arranged in four pairs of detector modules on each side of ATLAS at a distance of 240 m. Each detector module is made of ten layers of two times 64 scintillating fibres each (U and V planes). The fibres are coupled to 64 channels Multi-Anodes PhotoMultipliers Tubes read out by compact front-end electronics. The detector modules approach the LHC beam axis up to 1.5 mm to determine the luminosity from the differential spectrum of elastically scattered protons using the optical theorem. This Master thesis deals with the study of hit multiplicity, layer efficiency and spatial resolution of an ALFA prototype using the data obtained during the test beam performed in 2009 at Super Proton Synchrotron (SPS) of CERN. Zusammenfassung ALFA (Absolute Luminosity for ATLAS) ist ein hochpräziser Spurdetektor aus szin- tillierenden Fasern, der konzipiert wurde, um die absoluten Luminosität von LHC an der ATLAS Interaktionspunkt zu messen. Dieser Detektor besteht aus acht Roman Pots angeordnet in vier Paaren von Detektor-Module auf jeder Seite von ATLAS in einer Entfernung von 240 m. Jedes Detektor-Modul besteht aus szintillierenden Fasern, aufgeteilt auf 10 doppeltseitige Ebenen in U- und V-Geometrie. Die Fasern werden zu 64 Kanälen Multi-Anoden Photomultiplier Röhren gekoppelt und durch kompakte Front-End-Elektronik ausgelesen. Die Detektor-Module nähern sich zur Strahlachse von LHC bis 1.5 mm um aus dem differentiellen t-Spektrum der elastisch gestreuten Protonen mit der Benutzung des optischen Theorem, die Luminosität zu bestimmen. Diese Masterarbeit beschäftigt sich mit dem Studium der Hit Multipli- city, Effizienz der Ebenen und räumlichen Auflösung von einem Alfa-Prototyp. Die Analyse wurde mit den Daten des Test-Strahls in 2009 am Super Proton Synchrotron (SPS) des CERN durchgeführt. A mis padres, de quienes aprendí que las dificultades de la vida siempre conducen a felices logros ... i Contents 1 The LHC and its experiments 2 1.1 CERN . 2 1.2 Large Hadron Collider (LHC) . 2 1.2.1 Experiments at LHC . 2 1.3 ATLAS Experiment . 4 1.4 Forward Detectors for ATLAS . 8 2 Physics at LHC 12 2.1 Standard Model . 12 2.2 Soft Diffraction studies in ATLAS . 13 3 Luminosity 15 3.1 Methods to measure the luminosity . 15 3.2 Absolute luminosity determination . 17 3.2.1 Elastic scattering at small angles . 17 3.2.2 Luminosity determination from Coulomb nuclear interference . 18 3.3 Required beam optics properties . 19 3.3.1 An overview of the optics . 19 4 The ALFA method 23 4.1 Roman Pot stations . 23 4.1.1 ALFA scintillating fibre detector . 25 4.1.2 Overlap detectors . 27 4.2 ALFA readout electronics . 28 5 Set-up of test beam 32 5.1 Test beam conditions . 32 5.2 EUDET silicon pixel detector . 33 5.3 Conditions of data taking . 34 5.3.1 Data collection . 37 6 Data analysis 39 6.1 Hit multiplicity . 39 6.2 Layer efficiency . 43 6.3 Spatial Resolution . 46 7 Conclusions and outlook 49 ii List of Figures 1.1 Overall view of the LHC experiments . 3 1.2 Computer generated image of the whole ATLAS detector . 4 1.3 A schematic view of inner detector. 5 1.4 Computer Generated image of the ATLAS calorimeter. 7 1.5 Relative position of forward detectors for ATLAS. 8 1.6 LUCID detector. 9 1.7 Relative position of ZDC detectors to the Interaction Point . 10 1.8 Side view of the EM and hadronic ZDC modules . 11 2.1 Soft Diffractive Processes. 14 3.1 Lepton pair production in pp collisions: pp → p + `+`− + p . 16 3.2 Differential elastic cross section in dependence on momentum transfer t. 19 3.3 Quadrupole magnet in an accelerator. 20 3.4 Geometry of the parallel-to-point optics. 21 4.1 Schematic view of the positioning of the Roman Pots on each side of the ATLAS IP . 23 4.2 Schematic view of Roman Pot concept . 24 4.3 Roman Pot unit used for the test beam. 25 4.4 Scintillating fibres of ALFA detector . 26 4.5 Multi Anode Photomultiplier Tube (MAPMT) . 27 4.6 Front view of the arrangement of the fibres in main and overlap detector 28 4.7 Picture of Photo Multiplier Front-end (PMF) . 30 4.8 Readout system of PMF . 31 4.9 Block diagram of MAROC . 31 5.1 Geometry layout of the test beam setup. 32 5.2 EUDET telescope used in the test beam. 33 5.3 Charge spectrum from a MAMPT channel. 35 5.4 Plots for the gain equalization calibration. 35 5.5 The S-curve of the trigger efficiency for a MAPMT channel. 36 5.6 The S-curve of all channels of a PMF tube. 37 5.7 Tracks on the active areas of the lower and overlap detectors . 38 6.1 Hit multiplicity plots. 39 6.2 Mean hit multiplicity for each layer. 40 6.3 Simulated mean hit multiplicity. 41 6.4 Mean hit multiplicity as a function of the discriminator threshold. 41 6.5 Mean hit multiplicity plots as a function of gain factor and HV. 42 iii 6.6 Plots of track hits occupancy for U and V projections in ALFA 1 . 43 6.7 A reconstructed track for an event. 44 6.8 Layer efficiency of all layers in ALFA 1 . 44 6.9 Plots of track hits occupancy for U and V projections in ALFA 2 . 45 6.10 Layer efficiency of all layers in ALFA 2 . 45 6.11 Mean layer efficiency as a function of HV and gain factor. 46 6.12 Resolution determination using the two halves of ALFA. 47 6.13 Spatial resolution as a function of threshold signal. 48 6.14 Spatial resolution as a function of overlap cut . 48 iv List of Tables 1.1 Table of resolution and η coverage values for each ATLAS detector component. 5 2.1 Table of elementary particles with some of their main properties. 13 1 Chapter 1 The LHC and its experiments 1.1 CERN CERN, the European Organization for Nuclear Research, is the world’s largest centre for scientific research to study the basic constituents of matter. It is located in Geneva on the Swiss-French border and was created in 1954 by 12 Member States. Today, CERN has 20 Members States, 6 additional countries with observer status, Non-Member States as well as international organizations. 1.2 Large Hadron Collider (LHC) The Large Hadron Collider (LHC) is a two-ring superconducting hadron accelerator and collider built around 50 to 170 m underground in an existing circular tunnel of 27 km length at CERN so that it straddles the Swiss and French borders in the outskirts of Geneva. The tunnel was constructed between 1984 and 1989 for the CERN Large Electron-Positron Collider (LEP) machine, which operated until 2000 when it was removed to make way for the construction of LHC. The LHC√ is able to collide two counter rotating beams of protons at center of mass energy s = 14 TeV and has a design luminosity of 1034 cm−2s−1. The LHC will also collide heavy ions as lead nuclei, at 5.5 TeV per nucleon pair, but a design luminosity of 1027 cm−2s−1. The first collision at an energy of 3.5 TeV per beam took place in March 2010 with a luminosity of 1027 cm−2s−1. The LHC is composed of 1232 superconducting main dipoles of 15 m lenght used to guide the beams and 392 main quadrupole magnets of 7 m length used to focus the beam. They produce a magnetic field of 8.3 Tesla, the highest ever used in an accelerator, needed to reach 14 TeV energy collision. To achieve this, the winding cables inside thes magnets must be cooled to a temperature of 1.8 K to get the most efficient superconducting state without loss of energy. 1.2.1 Experiments at LHC There are six experiments at the LHC that are run by international collaborations, which are distinguished from each other by the characteristics of particle detector (Fig. 1.1). According to their size can be ordered as follows: ATLAS, CMS, ALICE, LHCb, TOTEM and LHCf. In what follows only the first four will be introduced. 2 3 Figure 1.1: The four main LHC experiments at CERN [1]. 1. CMS: The CMS (Compact Muon Solenoid) is, together with ATLAS, one of the largest LHC experiments. It has been built as a general-purpose detector to investigate a wide range of physics from Standard Model, such as searching the Higgs boson, to the physics beyond Standard Model, such as searching for extra dimensions and particles that could make up dark matter. CMS has its technical design based on a compact detector magnets capable to produce a magnetic field of 4 T. 2. ALICE: The heavy-ion detector ALICE (A Large Ion Collider Experiment) was de- signed to study the physics of strongly interacting matter at the TeV scale, where the formation of a new state of matter, the gluon plasma, is expected [2].
Details
-
File Typepdf
-
Upload Time-
-
Content LanguagesEnglish
-
Upload UserAnonymous/Not logged-in
-
File Pages61 Page
-
File Size-