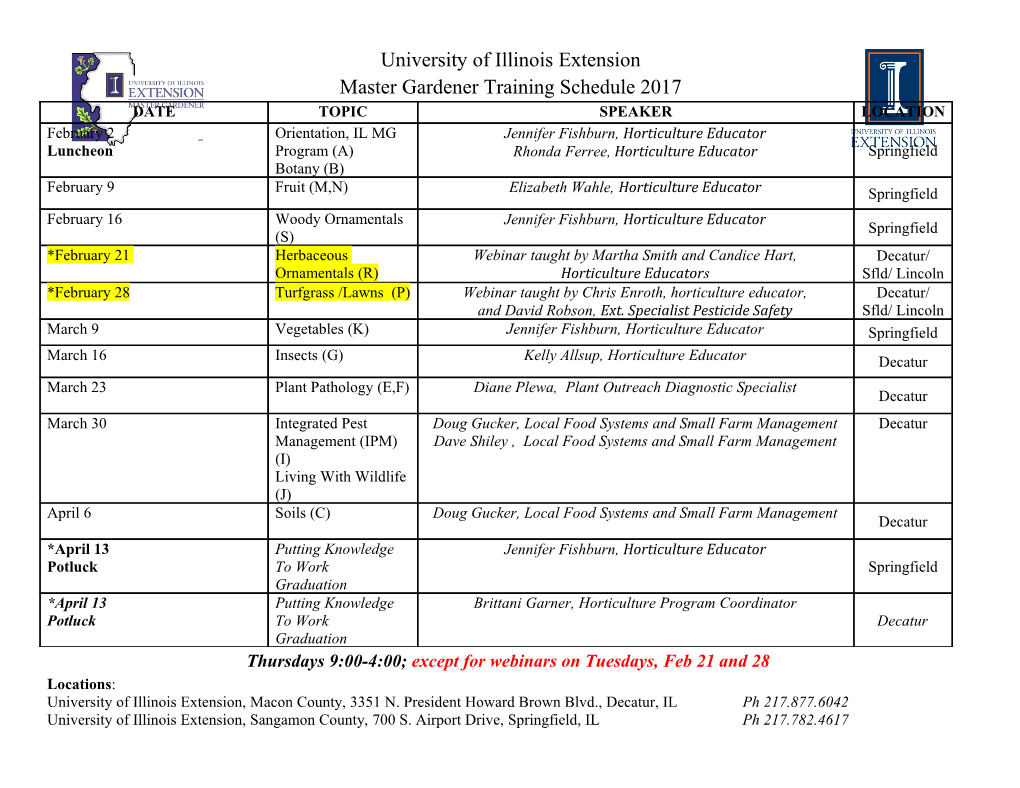
Ann. Geophys., 27, 877–883, 2009 www.ann-geophys.net/27/877/2009/ Annales © Author(s) 2009. This work is distributed under Geophysicae the Creative Commons Attribution 3.0 License. Velocity fluctuations in polar solar wind: a comparison between different solar cycles B. Bavassano, R. Bruno, and R. D’Amicis Istituto di Fisica dello Spazio Interplanetario, Istituto Nazionale di Astrofisica, Roma, Italy Received: 8 October 2008 – Revised: 26 January 2009 – Accepted: 4 February 2009 – Published: 20 February 2009 Abstract. The polar solar wind is a fast, tenuous and steady a dominant feature of the high-latitude heliosphere. Its prop- flow that, with the exception of a relatively short phase erties have been extensively investigated by Ulysses, the first around the Sun’s activity maximum, fills the high-latitude spacecraft with a highly inclined orbital plane with respect to heliosphere. The polar wind properties have been exten- the ecliptic. Launched in October 1990, the Ulysses’ out-of- sively investigated by Ulysses, the first spacecraft able to per- ecliptic journey began in February 1992, with a Jupiter grav- form in-situ measurements in the high-latitude heliosphere. ity assist. Presently data extend till December 2008, though The out-of-ecliptic phases of Ulysses cover about seventeen the spacecraft operations were expected to end in July 2008 years. This makes possible to study heliospheric properties (due to technical problems arisen in January 2008). With an at high latitudes in different solar cycles. In the present in- orbital period of 6.2 years, during this period of time Ulysses vestigation we focus on hourly- to daily-scale fluctuations of has covered two complete out-of-ecliptic orbits and ∼78% the polar wind velocity. Though the polar wind is a quite of the third one. However, during 2008 the data coverage uniform flow, fluctuations in its velocity do not appear negli- suffered a strong decline. gible. A simple way to characterize wind velocity variations Ulysses had its first polar passes in 1994 (South) and 1995 is that of performing a multi-scale statistical analysis of the (North), during the declining phase of solar cycle 22. In con- wind velocity differences. Our analysis is based on the com- trast, the second set of polar passes in 2000–2001 occurred putation of velocity differences at different time lags and the at high solar activity. During the 1994–1995 polar passes evaluation of statistical quantities (mean, standard deviation, Ulysses found an ordered heliosphere, with clear differences skewness, and kurtosis) for the different ensembles. The re- between the solar wind near the poles (polar wind) and that sults clearly show that, though differences exist in the three- around the equator (McComas et al., 2000). Near solar max- dimensional structure of the heliosphere between the inves- imum (2000–2001) things were more complex, making it tigated solar cycles, the velocity fluctuations in the core of hard to distinguish any particular region from another (Mc- polar coronal holes exhibit essentially unchanged statistical Comas et al., 2003). For the third set of polar passes in 2006– properties. 2008, during cycle 23, the solar activity was again low and Keywords. Interplanetary physics (MHD waves and turbu- the polar wind was again present at the high latitudes (McCo- lence; Solar wind plasma; Sources of the solar wind) mas et al., 2008). These new polar wind observations offer the opportunity of investigating, by comparison with those from the first orbit, if the polar wind properties vary between consecutive solar cycles, with reversal of the Sun’s magnetic 1 Introduction field polarity, namely along the ∼22-year Hale magnetic cy- cle. The polar solar wind is a fast plasma flow coming from po- As discussed by McComas et al. (2006, 2008), the helio- lar coronal holes. With the exception of a relatively short spheric structure during the declining phase of cycle 23 ex- phase around the Sun’s activity maximum, the polar wind is hibits significant differences with respect to that observed in cycle 22 at similar levels of solar activity. In fact, in cy- cle 23 polar coronal holes appear less well formed than usu- Correspondence to: B. Bavassano ally seen during minimum phases. This is probably the cause ([email protected]) of a more complicated structure of the current sheet, with a Published by Copernicus Publications on behalf of the European Geosciences Union. 878 B. Bavassano et al.: Polar wind velocity fluctuations V 1992 117 --1997 154 Table 1. Data intervals used to derive solar-rotation distributions of (km/s) V : start and end times (year, day, hour), minimum and maximum 800 distances (R, in AU), and latitudes (λ, in degrees, with n and s for 700 North and South, respectively). 600 interval time R λ 500 min max min max 400 South 1 1994 015 05 – 1994 356 05 1.61 – 3.76 49.7s – 80.2s South North North 1 1995 118 12 – 1996 094 22 1.44 – 3.59 41.3n – 80.2n 0o -30o -60o -90o -60o -30o 0o 30o 60o 90o 60o 30o 0o South 3 2006 165 17 – 2007 141 12 1.69 – 3.77 50.9s – 79.8s V 2004 254 --2008 347 North 3 2007 281 10 – 2008 257 06 1.50 – 3.58 42.3n – 79.8n (km/s) 800 700 600 be mainly related to conditions at the source solar region and to propagation effects. Changes in power spectra (Bavassano 500 et al., 2005) across the scales investigated here are indicative of the different nature of the velocity variations. Following 400 South North Burlaga and Forman (2002), to characterize these variations a multi-scale statistical analysis of the velocity differences 0o -30o -60o -90o -60o -30o 0o 30o 60o 90o 60o 30o 0o will be used. Fig. 1. Magnitude of the solar wind velocity V vs. latitude λ during 2 The analysed Ulysses data the first (top) and third (bottom) out-of-ecliptic orbit of Ulysses. Zero latitude at the left and right ends corresponds to the near- Figure 1 shows daily averages of solar wind velocity magni- aphelion solar equator crossing, that in the center is for the near- tude V vs. heliographic latitude λ during the first (top) and perihelion crossing. Blue and red horizontal segments indicate the third (bottom) out-of-ecliptic orbit. Zero values of latitude at analysed intervals (see text). The velocity decrease highlighted by green arrows in the bottom panel is caused by the tail of comet Mc- the left and right ends correspond to the solar equator cross- Naught. ing near the aphelion (5.41 AU, astronomical unit), while that in the center corresponds to the perihelion (1.34 AU) equato- rial crossing. Near the aphelion, only data at latitudes greater than 10◦, in absolute value, have been plotted. The curve larger average tilt and a non-planar shape. Hence, not sur- in the bottom panel ends with the latest available data at the prisingly, in the third orbit of Ulysses the polar wind ap- time of paper final version. Note that the velocity drop indi- pears less extended in latitude, slightly slower, less dense, cated by green arrows around the maximum southern latitude and cooler than in the first orbit (note that these trends lead to of the third orbit is caused by an encounter of Ulysses with a lower dynamic pressure of the wind in the third orbit). Mc- the comet McNaught tail (Neugebauer et al., 2007). Comas et al. (2008) have stressed that measurements in the Plasma measurements by Ulysses during its first out-of- near-ecliptic wind, while much more variable, match quanti- ecliptic orbit have provided the first in-situ observations of tatively with those by Ulysses and show essentially identical the polar solar wind (e.g., see Neugebauer, 1999; McCo- trends. Thus, the entire Sun appears involved in long-term mas et al., 2000). During the third orbit, as exhaustively changes of the solar wind output. discussed by McComas et al. (2008), the polar wind is con- Though the polar solar wind is a relatively steady flow, as fined to higher latitudes and has a lower velocity than in the compared to low latitude conditions, fluctuations in its veloc- first orbit. To get a complementary view of the solar wind ity magnitude V are not negligible. The goal of present anal- behaviour in the high-latitude heliosphere we have looked at ysis is of comparing, between cycles 22 and 23, the proper- wind velocity distributions on a solar rotation basis. The ex- ties of V variations at time scales between 1 h and ∼10 days. amined intervals are high-latitude cuts of the spacecraft orbit With this choice we cover fluctuations from scales at which with duration of thirteen solar rotations (as seen by Ulysses). a turbulent regime is typically developed in the wind up These intervals, drawn as blue lines in Fig. 1, are listed in to scales at which high-latitude microstreams (Neugebauer Table 1. The use of an integer number of solar rotations et al., 1995) become a dominant feature. In other words, at allows to cover all different conditions existing at the so- the short scales we are looking at variations mainly caused by lar surface without any bias. Note that, due to an asym- a turbulent cascade, while at the long scales variations should metry between the northern and southern orbital legs, the Ann. Geophys., 27, 877–883, 2009 www.ann-geophys.net/27/877/2009/ B. Bavassano et al.: Polar wind velocity fluctuations 879 F (%) 0 5 10 15 20 25 30 Table 2. Data intervals used for the multi-scale analysis of velocity 850 o South 1 -50 differences: start and end times (year, day, hour), minimum and 800 maximum distances (R, in AU), and latitudes (λ, in degrees, with n -60o V 750 and s for North and South, respectively).
Details
-
File Typepdf
-
Upload Time-
-
Content LanguagesEnglish
-
Upload UserAnonymous/Not logged-in
-
File Pages7 Page
-
File Size-