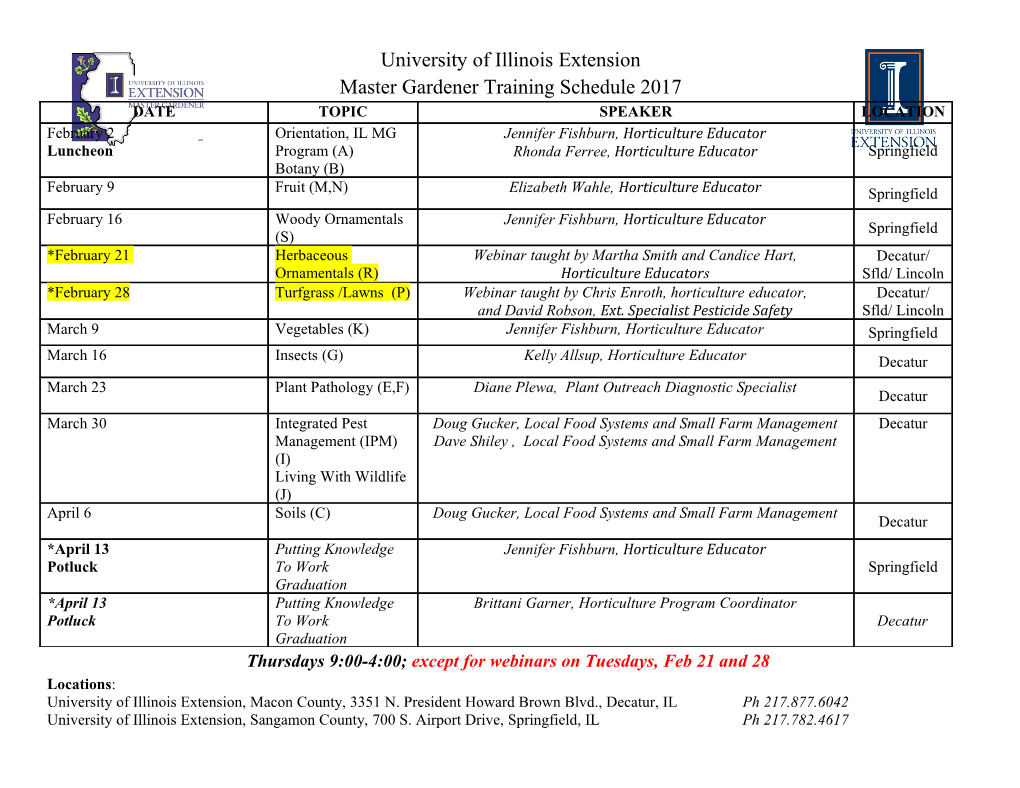
What is an isotope? A Nuclide Z X Z = atomic number = number of protons A = mass number = number of nucleons (protons + neutrons) N = neutron number = number of neutrons, i.e. N = A–Z The same Z – isotopes The same A – isobars Vojtěch Janoušek: Radiogenic isotope geochemistry Relative atomic mass • Dalton (or atomic mass unit - a.m.u.) and geochronology = 1/12 of the mass of 12C Periodic table of elements Radioactive decay D.I. Mendeleev Decay constant λ reflects the stability of atoms = what is the proportion of atoms that decay in given time t NNe 0 t D D0 Ne 1 Half-life t1/2 = how long it takes for half of the atoms to decay ln20693 . t 1 2 1 Types of radioactive decay Types of radioactive decay -β decay 87 Rb 87Sr 176 Lu 176 Hf 187 187 α decay Re Os 147Sm 143Nd +β decay Types of radioactive decay Example of branched decay Spontaneous fission 2 Example of decay chain (238U) Calculating age and initial ratio • Radioactive isotope (87Rb, 147Sm, ...) • Radiogenic isotope (87Sr, 143Nd, ...) • Stable isotope (86Sr, 144Nd, ...) • R (radioactive isotope to stable) e.g., (87Rb/86Sr) , (147Sm/144Nd) I (radiogenic isotope to stable) e.g., (87Sr/86Sr), (143Nd/144Nd) Calculating age and initial ratio Radiogenic/radioactive/stable isotopes t 143 143 I I i Re 1 Nd Nd 144 144 1 Nd Nd i 143 143 147 t ln 1 Nd Nd Sm t 147 144 144 144 e 1 Sm Nd Nd i Nd 144 Nd 87 87 87 Sr Sr Rb t 86 86 86 e 1 Sr Sr i Sr 176 Hf 176 Hf 176 Lu et 1 177 Hf 177 Hf 177 Hf i 1 I Ii 187 187 187 t ln 1 Os Os Re t R 186 186 186 e 1 Os Os i Os Treatise on Geochemistry kap. 3.08: Geochronology and etc. Thermochronology in Orogenic Systems (K. V. Hodges) 3 Rb–Sr method Sr isotopes Rubidium rubidium strontium 87Rb is –β radioactive: • Alkali, lithophile element Isotopic composition (%) λ = 1.42 . 10–11 yr–1 85Rb 72.1654 84Sr 0.56 87 87 0 • Substitutes for K Rb Sr 1e Q 87 86 (Steiger & Jäger 1977) (K-feldspars, micas, some clay Rb 27.8346 Sr 9.86 87 minerals, evaporites…) Sr 7.0 88 Sr 82.58 87 87 87 Sr Sr Rb t Relative atomic weight 86 86 86 e 1 Sr Sr i Sr Rb 85.46776 84Sr 83.9134 Strontium 86Sr 85.9092 • Alkaline earth; ion radius 87 87 87Sr 86.9088 Sr Sr somewhat greater than that 86 86 of Ca, substitutes for Ca 88Sr 87.9056 1 Sr Sr i t ln 87 1 (plagioclase, carbonates, apatite…), Rb sometimes also K (K-feldspars) 86 Sr • Rb is strongly incompatible element, thus its amount and Rb/Sr ratio rise with fractionation (esp. high in pegmatites) Rb–Sr method Isochrons Approx. blocking temperatures Possible applications Initial ratios are obtained for Rb–Sr system: 87 Sr 87 Sr • Cooling of plutonic rocks (blocking • Mineral lacking the radioactive element orthoclase 320 ºC 86 86 temperatures << solidus), crystallization of (e.g., apatite – contains nearly no Rb) 1 Sr Sr i biotite 350 ºC t ln 1 volcanic rocks (quick solidification) • Mineral extremely rich in radioactive element, 87 muscovite 450–500 ºC Rb soon dominated by the radiogenic component • Dating of mineralization (using cogenetic 86 Sr minerals – muscovite, biotite, adularia) Mezger (1990) (e.g. lepidolite – rich in Rb) Isochron method Appropriate material for Rb–Sr dating The samples define an isochron, if they: Mineral isochrons from plutonic rocks • are cogenetic (identical source – thus share the same initial ratios) o K-rich minerals (high Rb/Sr): K-feldspars (orthoclase, adularia), micas (muscovite, biotite, lepidolite), leucite • were contemporaneous (the same age) o Combined with Ca-rich minerals that are rather Rb poor (low Rb/Sr ratio): • formed closed isotopic system throughout plagioclase, apatite, ilmenite, amphibole, pyroxene their history Whole-rock samples • (there is a sufficient spread in compositions, i.e. in 87Rb/86Sr ratios) 4 Isochrons Sm–Nd method Samarium and neodymium samarium neodymium 87Sr 87 Sr 87 Rb t • Light Rare Earth Elements (LREE) Isotopic composition(%) 86 86 86 e 1 Sr Sr Sr • LREE form own minerals i 144Sm 3.1 142Nd 27.13 Biotite (monazite, allanite, bastnäsite); 147Sm 15.0 143Nd 12.18 •Substitute for Ca2+ a Th4+ in 148Sm 11.3 144Nd 23.80 y = a + bx rock-forming minerals (mainly 149Sm 13.8 145Nd 8.30 plagioclase, biotite, apatite) K-feldspar 150Sm 7.4 146Nd 17.19 • Incompatible elements, i.e. 152 148 Amphibole Sm 26.7 Nd 5.76 concentrations of Sm and Nd 154Sm 22.7 150Nd 5.64 increase with geochemical Plagioclase fractionation Geochronologically significant is decay of 147Sm: 147 143 1 Sm Nd intercept a slope b ~ age t lnb 1 Initial ratio 143 143 147 –12 –1 Nd Nd Sm t λ = 6.539 × 10 yr 144 144 144 e 1 (Lugmair & Marti 1978) Nd Nd i Nd Sm–Nd method Sm–Nd method Possible dating applications • Cooling of basic intrusions • Evolution of terrestrial Nd is explained by the model of a primitive mantle reservoir with Sm/Nd ratio of chondritic meteorites, so-called CHUR • Crystallization of basic volcanic rocks (rapid cooling) – (Chondritic Uniform Reservoir or Bulk Earth: DePaolo 1988) with present-day they are difficult to date by the Rb/Sr and U/Pb methods (especially if very old, partly altered and do not contain zircon) isotopic composition: 147 144 • Dating high-grade metamorphism Sm/ NdCHUR = 0.1967 143 144 (granulite- and eclogite-facies) Nd/ NdCHUR = 0.512638 (Jacobsen & Wasserburg 1980) Appropriate material for Sm–Nd dating Minerals from (ultra-)basic igneous rocks SA pyroxene, amphibole, plagioclase, ilmenite, apatite, 143Nd monazite, zircon… 144 143 144 i Nd 4 Garnet (+ Cpx) in metamorphic rocks Small differences in Nd/ Nd i 110 Nd CHUR Whole-rock samples ratios → initial composition of 143Nd the Nd is normally expressed 144 using the ε notation Nd Nd i Where: index „i“ denotes initial ratio, SA = sample 5 Nd isotopes Nd model ages trivalent REE – lanthanide Sm/Nd decreases during differentiation , Model age = moment in the past contraction, Nd shows greater ionic thus mantle develops higher 143Nd/144Nd when Nd isotopic composition of the radius than Sm and thus the ratios than crust sample was identical with that of some Sm/Nd decrease during geochemical model reservoir (most often CHUR or fractionation; this fractionation is still Depleted Mantle — DM). comparably limited Parcial melting of CHUR Melt — depleted in Sm, Sm/Nd ratio is lower than that of CHUR Residue — enriched in Sm, higher Sm/Nd ratio The melt-depleted mantle domains develop, over time, 143Nd/144Nd greater than CHUR (so-called Depleted Mantle, DM – DePaolo 1988) Isotopic provinces of the Western U.S. based on crustal residence times (ΤDM) (Bennett and DePaolo 1987) Lu–Hf method Lu–Hf method Lutetium lutetium hafnium Appropriate material for Lu–Hf dating • The heaviest among REE Isotopic composition (%) • Usage analogous to the Sm–Nd method • Enters the crystal structure of • Shorter half life and higher Lu/Hf ratios of common rocks and minerals result many accessories: allanite, 175Lu 97.4 174Hf 0.16 in greater variability of the Hf isotopic composition (= advantage) monazite, apatite and titanite 176Lu 2.6 176Hf 5.2 177Hf 18.6 178 Hafnium Hf 27.1 Mineral phases in igneous and metamorphic rocks 179Hf 13.7 • Transition metal 180Hf 35.2 • High Lu/Hf: titanite, apatite, monazite, allanite • Geochemical behaviour strongly •LowLu/Hf: zircon = ideal mineral for Hf isotopic studies: resembles Zr, for which it readily substitutes in accessory minerals – Hf substitutes into the crystal lattice (and is thus relatively immobile) (zircon) – High concentrations of Hf and low Lu/Hf ratios in zircon require (almost) 176 • Lu shows branched decay: no age correction for the initial ratios 1 -β emission l = 1.94 × 10–11 yr –1 – Is often combined with in situ U–Pb dating, (97 % of decays) mostly using LA ICP-MS 176 Lu 176 Hf (Patchett & Tatsumoto 1980) Whole-rock samples 2 Electron capture (3 % of decays) no geochronological meaning • Acid as well as basic (Lu/Hf ratio decreases with geochemical fractionation) 6 Lu–Hf method Lu–Hf method Petrogenesis of igneous rocks Small differences in 176Hf/177Hf → initial ratios of Hf isotopes are usually expressed as: Isotopic evolution of Hf in a chondritic reservoir, in an igneous rock, formed by its partial melting, and in residue of this partial melting 176 SA (Faure 1986) Hf 177 i Hf i 4 Hf 110 176 CHUR Hf 177 Hf i Where: index „i“ = initial ratio, SA = sample, CHUR = Chondritic Uniform Reservoir εHf < 0: rock originated from (or assimilated significant amount of) material with Lu/Hf lower than CHUR (e.g., old crustal rocks). εHf > 0: rock comes from a source with high Lu/Hf (e.g. mantle domains impoverished in incompatible elements due to previous partial melting – 176 177 176 177 Lu/ HfCHUR = 0.0334 a Hf/ HfCHUR = 0.28286 Depleted Mantle = DM) (Patchett & Tatsumoto 1980) K–Ar and Ar–Ar methods K–Ar and Ar–Ar methods Potassium potassium argon • Alkali metal 40K is radioactive with branched decay Isotopic composition (%) • Lithophile element th 1. -β emission (88.8 % of decays) (8 most common 39K 93.2581 36Ar 0.337 in the Earth‘s crust) 40 38 K 0.01167 Ar 0.063 40 40 0 –10 –1 K Ca e λβ = 4.962 . 10 yr • Three naturally occurring 41K 6.7302 40Ar 99.600 1 39 40 41 isotopes: K, K, K Relative atomic mass 2.
Details
-
File Typepdf
-
Upload Time-
-
Content LanguagesEnglish
-
Upload UserAnonymous/Not logged-in
-
File Pages15 Page
-
File Size-