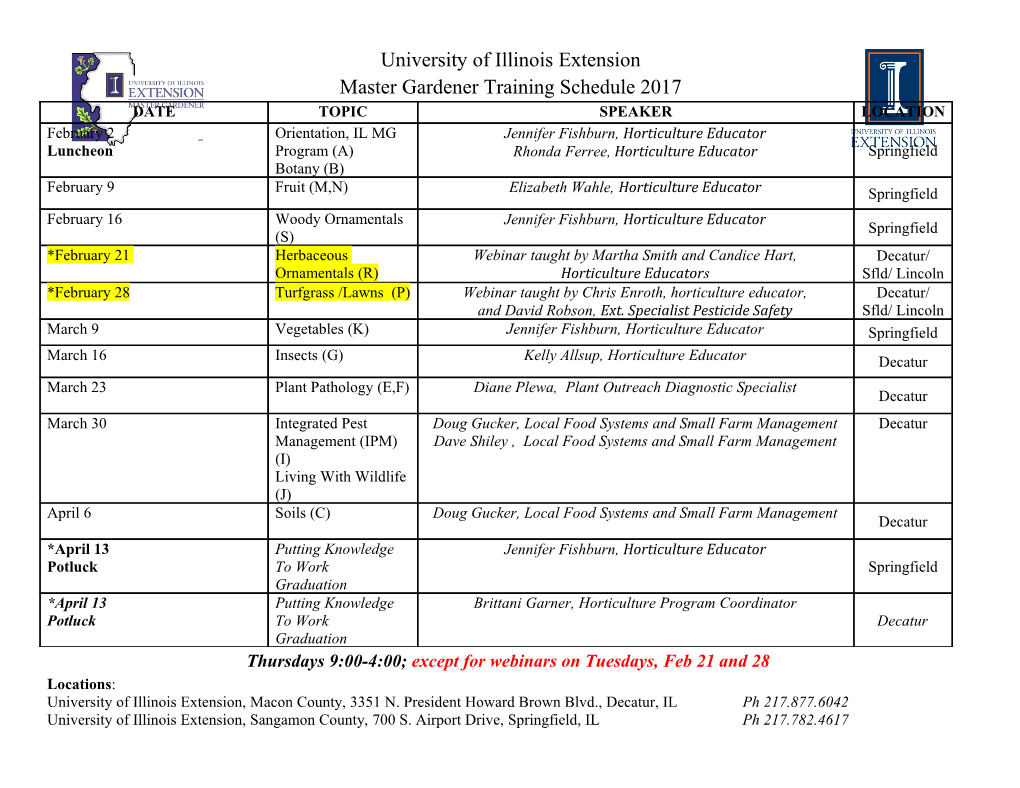
To be published in the Astronomical Journal, June 1999 Using Hubble Space Telescope Imaging of Nuclear Dust Morphology to Rule Out Bars Fueling Seyfert Nuclei1 Michael W. Regan2,3 Carnegie Institution of Washington, Department of Terrestrial Magnetism, 5241 Broad Branch Road, Washington, DC 20015 John S. Mulchaey4 The Observatories of the Carnegie Institution of Washington, 813 Santa Barbara Street, Pasadena, CA 91101 ABSTRACT If AGN are powered by the accretion of matter onto massive black holes, how does the gas in the host galaxy lose the required angular momentum to approach the black hole? Gas easily transfers angular momentum to stars in strong bars, making them likely candidates. Although ground-based searches for bars in active galaxies using both optical and near infrared surface brightness have not found any excess of bars relative to quiescent galaxies, the searches have not been able to rule out small-scale nuclear bars. To look for these nuclear bars we use HST WFPC2-NICMOS color maps to search for the straight dust lane signature of strong bars. Of the twelve Seyfert galaxies in our sample, only three have dust lanes consistent with a strong nuclear bar. Therefore, strong arXiv:astro-ph/9903053v1 3 Mar 1999 nuclear bars cannot be the primary fueling mechanism for Seyfert nuclei. We do find that a majority of the galaxies show an spiral morphology in their dust lanes. These spiral arms may be a possible fueling mechanism. Subject headings: galaxies: Seyfert — galaxies: ISM — galaxies: nuclei 1Based on observations with the NASA/ESA Hubble Space Telescope, obtained at the Space Telescope Science Institute, which is operated by the Association of Universities for Research in Astronomy, Inc. under NASA contract No. NAS5-26555 [email protected] 3Hubble Fellow [email protected] –2– 1. Introduction One of the goals of AGN research is to try to understand what transports the gas from the large host galaxy scales (∼1-10kpc) down close enough to the nucleus (<1 pc) to fuel an AGN. To accomplish this fueling the gas must decrease its angular momentum by several orders of magnitude. How does the gas lose its angular momentum? One possibility is that gas could lose angular momentum to stars in the shocks and gravitational torques caused by a bar-like gravitational potential (Sholsman, Frank, & Begelman 1989). If this is true, we might expect there to be more bars in active galaxies than in quiescent galaxies. Surveys have looked for this excess of bars and none has been found (Kotilainen et al. 1992; Zitelli et al. 1993; McLeod & Reike 1995; Alonso-Herrero, Ward, & Kotilainen 1996; Mulchaey & Regan 1997; Ho, Filippenko, & Sargent 1997). The lack of observational evidence for an excess of bars in these survey has not ruled out bars as a mechanism of fueling active galaxies for several reasons. One possibility is that there is an inherent difference between active galaxies and normal galaxies in that not all normal galaxies may contain a massive black hole. In this case the lack of an excess of bars in Seyfert galaxies compared to normal galaxies would not rule out bars as a fueling mechanism. However, recent studies of quiescent galaxies using both ground-based observations and the HST have revealed kinematic evidence for the presence of black holes in almost every galaxy studied (Kormendy & Richstone 1995). Another possibility is that, due to a lack of resolution, all studies to date have been unable to detect small scale bars (<∼3′′), which are all that is necessary to provide fuel to an AGN. Almost all studies of AGN fueling in the past have focused on looking for non- axisymmetric perturbations in the stellar surface density under the assumption that these lead to perturbations in the gravitational potential. However, this may not be the best way to search for small-scale nuclear bars. Even in the near infrared the stellar surface density is subject to contamination by dust extinction and regions of current star formation. This contamination makes it hard to determine the gravitational potential from the stellar surface density. Since the fuel for AGN is provided by the interstellar medium (ISM), another avenue of investigation is to study the ISM directly. Because the ISM in spiral galaxies is dynamically cold, it responds strongly to perturbations in the gravitational potential. If we can characterize the morphology of the ISM, it could provide a more sensitive probe of the gravitational potential than the stellar surface density morphology. To search for the signatures of a nuclear bar using the ISM morphology requires the resolution of the Hubble Space Telescope (HST). In this paper we present our observations of Seyfert galaxies made with the Near Infrared Camera and Multi-object Spectrometer (NICMOS). We combine the NICMOS observations with archival observations of the same –3– galaxies made with the Wide Field and Planetary Camera 2 (WFPC2) to form color maps. We use these color maps to investigate the nuclear ISM morphology of a set of Seyfert galaxies as a test of whether small scale bars fuel Seyfert nuclei. 2. Using the ISM as a Probe of the Gravitational Potential Since stars compose the vast majority of the mass in the central regions of disk galaxies, it would make sense to search for perturbations in the gravitational potential by looking at the stellar surface density. The problem is that the stars in the old stellar disk have velocity dispersions of ∼40 km s−1 (Mihalas & Binney 1981) which will tend to wash out the effects of perturbations. To complicate matters, in the nuclear regions of galaxies the velocity dispersion of the spheroid component is >100 km s−1 leading to even more smoothing of the surface density variations. The low velocity dispersion of the ISM (∼4 km s−1 in the local disk of the Milky Way (Combes 1991)) will lead to much larger surface density perturbations, making it a better probe of the gravitational potential. To quantify the advantage of using the ISM surface density, we can compare the surface density variations of the two components using a model galaxy. For our model galaxy we determine the stellar surface density by adding the contributions of three mass components: a disk, a bulge, and a bar. The surface density of the Kuzmin-Toomre disk component, σd, is 2 2 2 −1.5 σd(r)=(v0/2πGr)(1 + r /r0) , (1) −1 where v0 = 164 km s and r0 = 20 kpc. The volume density of the bulge, ρb, is 2 2 −1.5 ρb = ρcen(1 + r /rcore) , (2) where ρcen is the central density of the bulge and rcore is the core radius of the bulge. We use a Ferrers ellipsoid for the bar density with a density, ρbar, of 2 ρbar = ρ0(1 − g ) forg < 1 (3) and ρbar =0 forg >=1, where g2 = y2/a2 +(x2 + z2)/b2 and a and b are the semi-major and semi-minor axis, respectively. To convert the volume densities into observable units we integrate the volume densities of the bar and bulge over ± 10 kpc in the z direction to form surface densities. We then sum the three surface density components to give the total surface density (Figure 1a). –4– Fig. 1.— a) The model stellar surface density of barred galaxy. b) The model gas surface density of a barred galaxy. Notice how the dust lanes along the leading edge of the bar are straight. This is the signature of a strongly barred galaxy. We use the hydrodynamic models of gas flow in a barred potential from Piner, Stone & Teuben (1995) to find the gas surface density for this mass surface density. The resulting gas surface density is shown in Figure 1b. The peak variation in the azimuthal gas surface density occurs at a radius of ∼2 kpc. At this radius the azimuthal gas surface density variation is ∼20 to 1. At that same radius in the stellar surface density (Figure 1a) the peak density variation with azimuth is ∼2.2 to 1. Therefore, at a given level of sensitivity to azimuthal surface density variations, the ISM surface density will reveal much smaller non-axisymmetric perturbations than the stellar surface density. Detecting the ISM surface density variations at sub-arcsecond resolution cannot be done with conventional methods of observing the ISM in galaxies such as millimeter interferometers and thermal infrared observations of dust. Although the current generation of millimeter arrays do have sub-arcsecond resolution, they will not have the surface brightness sensitivity to detect normal molecular clouds at this resolution until the arrival of the Millimeter Array. Thermal infrared observations by ISO of hot dust in Centaurs A did reveal a the expected dust morphology of a strong bar (Mirabel et al. 1999) but the resolution of these observations was 3′′which will only resolve the dust morphology in relatively nearby galaxies. An alternative method for observing the morphology of the ISM, is to look for the dust component of the ISM in extinction. One problem with using –5– dust extinction as a tracer of the ISM is that if the extinction is high enough for the dust to become optically thick then the light will be dominated by the foreground unreddened stars. This leads to regions with large amounts of dust having relatively unreddened colors. This problem can be minimized by using a near infrared color as the long wavelength color, which will provide a much larger dynamic range of dust column depths that will be detectable in a extinction map. Another advantage of using the dust morphology to probe the potential instead of the stellar surface density is that the problem of the the stellar isophotes being influenced by the presence of dust or regions of current star formation is avoided.
Details
-
File Typepdf
-
Upload Time-
-
Content LanguagesEnglish
-
Upload UserAnonymous/Not logged-in
-
File Pages25 Page
-
File Size-