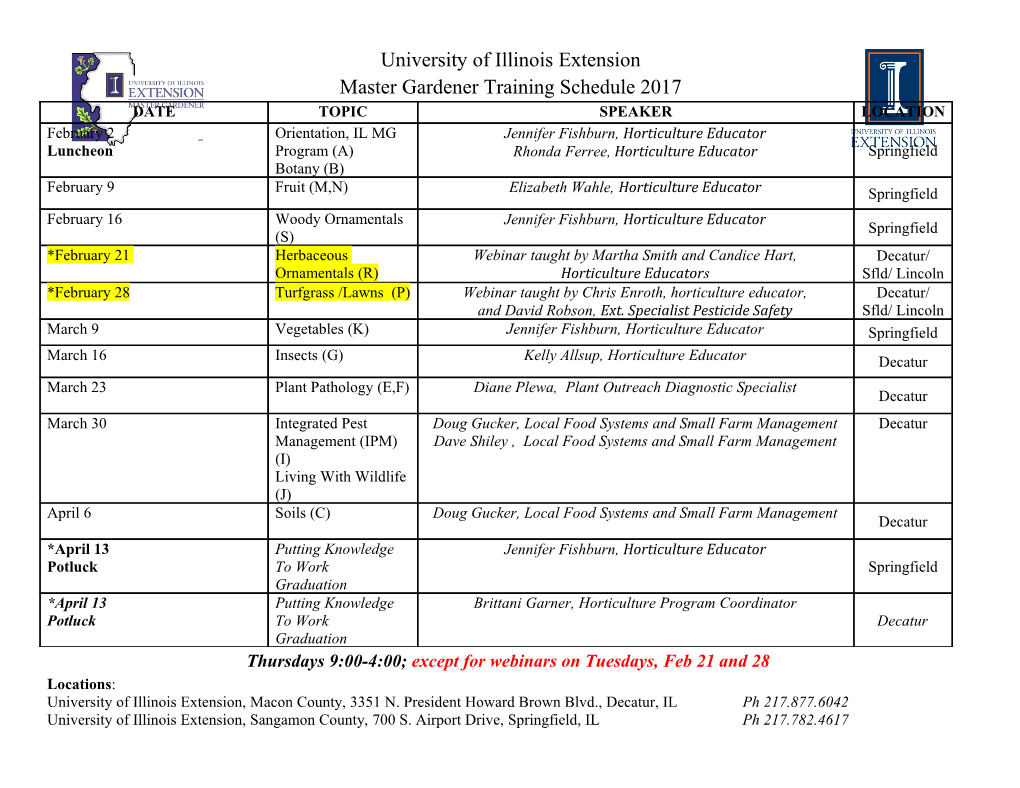
Formulas in Solid Mechanics Tore Dahlberg Solid Mechanics/IKP, Linköping University Linköping, Sweden This collection of formulas is intended for use by foreign students in the course TMHL61, Damage Mechanics and Life Analysis, as a complement to the textbook Dahlberg and Ekberg: Failure, Fracture, Fatigue - An Introduction, Studentlitteratur, Lund, Sweden, 2002. It may be use at examinations in this course. Contents Page 1. Definitions and notations 1 2. Stress, Strain, and Material Relations 2 3. Geometric Properties of Cross-Sectional Area 3 4. One-Dimensional Bodies (bars, axles, beams) 5 5. Bending of Beam Elementary Cases 11 6. Material Fatigue 14 7. Multi-Axial Stress States 17 8. Energy Methods the Castigliano Theorem 20 9. Stress Concentration 21 10. Material data 25 Version 03-09-18 1. Definitions and notations Definition of coordinate system and loadings on beam Mz qx( ) My Tz N Mx Ty x M N y Ty x T z A z M y M L z Loaded beam, length L, cross section A, and load q(x), with coordinate system (origin at the geometric centre of cross section) and positive section forces and moments: normal force N, shear forces Ty and Tz, torque Mx, and bending moments My, Mz Notations Quantity Symbol SI Unit Coordinate directions, with origin at geometric centre of x, y, z m cross-sectional area A σ 2 Normal stress in direction i (= x, y, z) i N/m τ 2 Shear stress in direction j on surface with normal direction i ij N/m ε Normal strain in direction i i τ γ Shear strain (corresponding to shear stress ij) ij rad Moment with respect to axis iM, Mi Nm Normal force N, P N (= kg m/s2) Shear force in direction i (= y, z) T, Ti N Load q(x) N/m Cross-sectional area A m2 Length L, L0 m Change of length δ m Displacement in direction xu, u(x), u(x,y)m Displacement in direction yv, v(x), v(x,y)m Beam deflection w(x)m 4 Second moment of area (i = y, z) I, Ii m Modulus of elasticity (Young’s modulus) E N/m2 Poisson’s ratio ν Shear modulus G N/m2 Bulk modulus K N/m2 Temperature coefficient α K− 1 1 2. Stress, Strain, and Material Relations σ Normal stress x ∆ ∆N = fraction of normal force N σ = N σ = N x or x lim ∆ A ∆A → 0 ∆A A = cross-sectional area element τ Shear stress xy (mean value over area A in the y direction) T τ = y (= τ ) xy A mean ε Normal strain x δ Linear, at small deformations ( << L0) δ ( ) δ = change of length ε = ε = du x x or x L = original length L0 dx 0 u(x) = displacement Non-linear, at large deformations δ L L = actual length (L = L0 + ) ε = x ln L0 γ Shear strain xy ∂ ( , ) ∂ ( , ) γ = u x y + v x y xy ∂y ∂x Linear elastic material (Hooke’s law) Tension/compression σ ∆T = change of temperatur ε = x +α∆T x E Lateral strain ε =−νε y x Shear strain τ γ = xy xy G Relationships between G, K, E and ν E E G = K = 2 ( 1 +ν) 3 ( 1 − 2ν) 2 3. Geometric Properties of Cross-Sectional Area e The origin of the coordinate system Oyz is O y at the geometric centre of the cross section f dA z Cross-sectional area A ⌠ dA = area element A = dA ⌡A Geometric centre (centroid) ⌠ e = ζ = distance from η axis to geometric e ⋅ A = ζ dA gc ⌡A centre f = η distance from ζ axis to geometric ⌠ gc f ⋅ A = η dA centre ⌡A First moment of area = ⌠ = ⌠ A’ = the “sheared” area (part of area A) Sy zdA and Sz ydA ⌡A’ ⌡A’ Second moment of area = ⌠ 2 Iy = second moment of area with respect to Iy z dA ⌡A the y axis = ⌠ 2 Iz = second moment of area with respect to Iz y dA ⌡A the z axis = ⌠ Iyz = second moment of area with respect to Iyz yzdA ⌡A the y and z axes Parallel-axis theorems First moment of area ⌠ ⌠ Sη = (z + e) dA = eA and Sζ = (y + f) dA = fA ⌡A ⌡A Second moment of area = ⌠ ( + )2 = + 2 , = ⌠ ( + )2 = + 2 , Iη z e dA Iy e A Iζ y f dA Iz f A ⌡A ⌡A = ⌠ ( + )( + ) = + Iηζ z e y f dA Iyz efA ⌡A 3 Rotation of axes y Coordinate system Ωηζ has been rotated α z the angle with respect to the coordinate system Oyz y d A z = ⌠ ζ2 = 2 α+ 2 α− α α Iη dA Iy cos Iz sin 2Iyz sin cos ⌡A = ⌠ η2 = 2 α+ 2 α+ α α Iζ dA Iy sin Iz cos 2Iyz sin cos ⌡A I − I = ⌠ ζη =( − ) α α+ ( 2 α− 2 α)= y z α+ α Iηζ dA Iy Iz sin cos Iyz cos sin sin 2 Iyz cos2 ⌡A 2 Principal moments of area I + I I − I 2 I = y z ± R where R = y z + I 2 1,2 2 √ 2 yz I1 + I2 = Iy + Iz Principal axes − I I − I A line of symmetry is always a principal sin 2α= yz or cos 2α= y z R 2R axis Second moment of area with respect to axes through geometric centre for some symmetric areas (beam cross sections) B Rectangular area, base B, height H y H BH3 HB3 I = and I = y 12 z 12 z Solid circular area, diameter D y D πD 4 I = I = y z 64 z Thick-walled circular tube, diameters D y d D and d π I = I = ( D 4 − d 4 ) z y z 64 4 Thin-walled circular tube, radius R and R t y wall thickness t (t << R) = =π 3 Iy Iz R t z Triangular area, base B and height H H BH3 HB3 y I = and I = B/ 2 B/ 2 y 36 z 48 z a a a Hexagonal area, side length a 5√3 y I = I = a 4 a a y z 16 z 2a Elliptical area, major axis 2a and minor axis 2b y 2 b πab3 πba3 I = and I = z y 4 z 4 Half circle, radius a (geometric centre at e) a π y 8 4 4 4a e I = − a ≅ 0, 110 a and e = y 8 9π 3π z 4. One-Dimensional Bodies (bars, axles, beams) Tension/compression of bar Change of length NL N, E, and A are constant along bar δ= or EA L = length of bar ⌠ L ⌠ L N(x) N(x), E(x), and A(x) may vary along bar δ= ε(x)dx = dx ⌡0 ⌡0 E(x)A(x) Torsion of axle Maximum shear stress M Mv = torque = Mx τ = v max Wv = section modulus in torsion (given Wv below) Torsion (deformation) angle M L Mv = torque = Mx Θ= v Kv = section factor of torsional stiffness GKv (given below) 5 Section modulus Wv and section factor Kv for some cross sections (at torsion) Torsion of thin-walled circular tube, radius R t y R, thickness t, where t << R, = π 2 = π 3 Wv 2 R tKv 2 R t z (s) Thin-walled tube of arbitrary cross section A = area enclosed by the tube t(s) s t(s) = wall thickness Area A s = coordinate around the tube 2 = = 4A Wv 2Atmin Kv ⌠ − [t(s)] 1 ds ⌡s Thick-walled circular tube, diameters D y d D and d, π D 4 − d 4 π W = K = (D 4 − d 4) z v 16 D v 32 Solid axle with circular cross section, y D diameter D, πD 3 πD 4 W = K = z v 16 v 32 Solid axle with triangular cross section, a side length a y a 3 a 4 √3 a/2 a/2 W = K = z v 20 v 80 2a Solid axle with elliptical cross section, major axle 2a and minor axle 2b y 2 b π π 3 3 = 2 = a b z Wv ab Kv 2 a 2 + b 2 b Solid axle with rectangular cross section b by a, where b ≥ a y a = 2 = 3 Wv kWv a bKv kKv a b z for kWv and kKv, see table below 6 Factors kWv and kKv for some values of ratio b / a (solid rectangular cross section) b / akWv kKv 1.0 0.208 0.1406 1.2 0.219 0.1661 1.5 0.231 0.1958 2.0 0.246 0.229 2.5 0.258 0.249 3.0 0.267 0.263 4.0 0.282 0.281 5.0 0.291 0.291 10.0 0.312 0.312 ∞ 0.333 0.333 Bending of beam Relationships between bending moment My = M(x), shear force Tz = T(x), and load q(x)on beam dT(x) dM(x) d2M(x) =−q(x), = T(x), and =−q(x) dx dx dx 2 Normal stress I (here I ) = second moment of area (see σ=N + Mz y A I Section 12.2) Maximum bending stress | M| I |σ| = where W = max b | | W = section modulus (in bending) Wb z max b Shear stress TS SA’ = first moment of area A’ (see Section τ= A’ Ib 12.2) b = length of line limiting area A’ τ τ =µT gc = shear stress at geometric centre gc A µ = the Jouravski factor The Jouravski factor µ for some cross sections rectangular 1.5 triangular 1.33 circular 1.33 thin-walled circular 2.0 elliptical 1.33 ideal I profile A / Aweb 7 Skew bending Axes y and z are not principal axes: M (zI − yI )−M (yI − zI ) σ=N + y z yz z y yz − 2 Iy, Iz, Iyz = second moment of area A Iy Iz Iyz Axes y’ and z’ are principal axes: M z’ M y’ σ=N + 1 − 2 I1, I2 = principal second moment of area A I1 I2 Beam deflection w(x) Differential equations d2 d2 EI(x) w(x) = q(x) dx 2 dx 2 when EI(x) is function of x d4 EI w(x)=q(x) dx 4 when EI is constant Homogeneous boundary conditions Clamped beam end x x=L d w(*)=0 and w(*)=0 dx where * is the coordinate of beam end (to be entered after differentiation) Simply supported beam end x x=L d2 w(*)=0 and − EI w(*)=0 dx 2 Sliding beam end x x=L d d3 w(*)=0 and − EI w(*)=0 dx dx 3 Free beam end x x=L d2 d3 − EI w(*)=0 and − EI w(*)=0 dx 2 dx 3 8 Non-homogeneous boundary conditions (a) Displacement δ prescribed w(*)=δ x x=L (a) Θ (b) Slope prescribed O d w(*)=Θ (b) dx z O M00x x=L M (c) Moment M0 prescribed d2 (c) − EI w(*)=M dx 2 0 P x x=L P (d) Force P prescribed (d) d3 − EI w(*)=P dx 3 Beam on elastic bed Differential equation d4 EI w(x)+kw(x)=q(x) dx 4 EI = constant bending stiffness k = bed modulus (N/m2) Solution ( )= ( )+ ( ) w x wpart x whom x where λ −λ k w (x)={C cos (λx)+C sin (λx)} e x +{C cos (λx)+C sin (λx)} e x ; λ4 = hom 1 2 3 2 4EI Boundary conditions as given above Beam vibration Differential equation ∂4 ∂2 EI = constant bending stiffness EI w(x, t)+m w(x, t)=q(x, t) ∂x 4 ∂t 2 m = beam mass per metre (kg/m) t = time Assume solution w(x,t)=X(x)⋅T(t).
Details
-
File Typepdf
-
Upload Time-
-
Content LanguagesEnglish
-
Upload UserAnonymous/Not logged-in
-
File Pages26 Page
-
File Size-