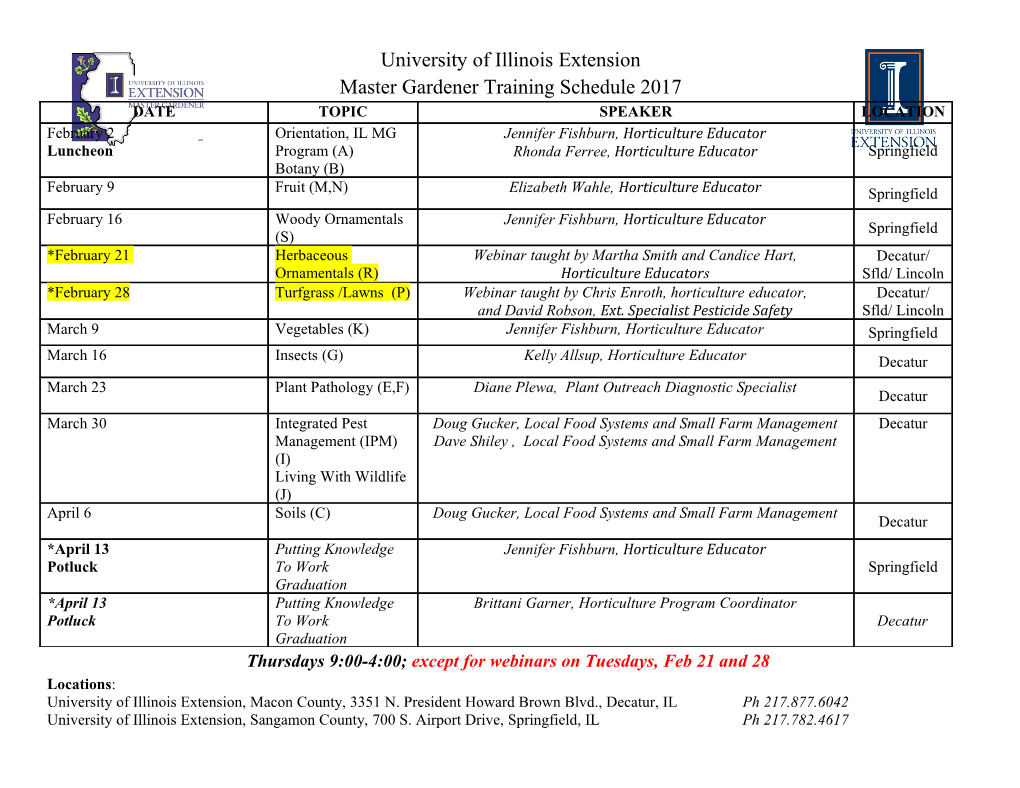
REPORT FHWA/NY/SR-04/142 Estimating Fatigue Life of Patroon Island Bridge Using Strain Measurements RYAN LUND SREENIVAS ALAMPALLI SPECIAL REPORT 142 TRANSPORTATION RESEARCH AND DEVELOPMENT BUREAU NEW YORK STATE DEPARTMENT OF TRANSPORTATION George E. Pataki, Governor/Joseph H. Boardman, Commissioner ESTIMATING FATIGUE LIFE OF PATROON ISLAND BRIDGE USING STRAIN MEASUREMENTS Ryan Lund, Civil Engineer I, Transportation Research & Development Bureau Sreenivas Alampalli, Director, Bridge Program and Evaluation Services Bureau Special Report 142 November 2004 TRANSPORTATION RESEARCH AND DEVELOPMENT BUREAU New York State Department of Transportation, 50 Wolf Road, New York 12232 ABSTRACT The design fatigue life of a bridge component is based on the stress spectrum the component experiences and the fatigue durability. Changes in traffic patterns, volume, and any degradation of structural components can influence the fatigue life of the bridge. A fatigue life evaluation, reflecting the actual conditions, has value to bridge owners. This report presents a study where the remaining fatigue life of the Patroon Island Bridge, which carries Interstate 90 over the Hudson River, was estimated as part of a structural integrity evaluation and a larger evaluation of the entire interchange. The Patroon Island Bridge consists of ten spans. Seven spans are considered the main spans and consist of steel trusses and concrete decks. The other three spans are considered approach spans and consist of plate girders. The overall bridge length is 1,795 feet. Procedures outlined in the AASHTO Guide Specifications for Fatigue Evaluation of Existing Steel Bridges and strain data from critical structural members were used to estimate the remaining fatigue life of selected bridge components. The results indicate that most of the identified critical details have an infinite remaining safe fatigue life and others have a substantial fatigue life, however the remaining fatigue life has not been determined for damaged and cracked members. iii CONTENTS I. INTRODUCTION ..........................................................1 A. Background ............................................................1 B. Current Status of the Bridge................................................4 C. Study Objectives ........................................................6 II. INSTRUMENTATION AND DATA COLLECTION ..............................7 A. Gage Locations .........................................................7 B. Instrumentation ........................................................11 C. Data Collection and Analysis..............................................12 III. EFFECTIVE STRESSES FOR CRITICAL DETAILS ............................19 IV. FATIGUE LIFE EVALUATION .............................................25 A. Detail Classification .....................................................25 B. Infinite Fatigue Life Check................................................26 C. Finite Remaining Fatigue Life .............................................28 V. CONCLUSIONS ..........................................................31 ACKNOWLEDGMENTS ......................................................33 REFERENCES ..............................................................35 APPENDICES Appendix A: Histograms ...................................................37 v I. INTRODUCTION The remaining fatigue life of bridges can play a major role in making cost-effective decisions regarding rehabilitation versus replacement of existing bridges, especially for those bridges that are part of a busy interchange. The design fatigue life of a bridge is generally based on the truck traffic data, prevalent specifications, and the fatigue durability of the components in the bridge. The actual truck traffic volume that a bridge experiences, and is expected to experience for the design life of the bridge, will influence the fatigue life of the bridge components. An evaluation based on actual conditions can benefit bridge owners. The remaining safe fatigue life of the Patroon Island Bridge in New York State was evaluated as part of a structural integrity evaluation of a major interchange (1). Procedures outlined in the AASHTO Guide Specifications for Fatigue Evaluation of Existing Steel Bridges and strain data from critical structural members were used to estimate the remaining fatigue life of selected bridge components (2, 5). A rain-flow algorithm (3,4) and Miner's Rule (2) were used as part of the analysis. The analysis showed that most of the members analyzed have an infinite fatigue life, however the remaining fatigue life has not been determined for damaged and cracked members. In this report the remaining safe life of structural details is determined as opposed to the remaining mean life. There is a 97.7% and 99.9% probability that the actual fatigue life will exceed the remaining safe life for redundant and non redundant details, respectively (2). A. BACKGROUND The Patroon Island Bridge, as illustrated in Figures 1 and 2, is part of a major interchange in Albany, New York. The bridge carries Interstate 90, in the east-west direction, over the Hudson River. The bridge consists of ten spans. Seven spans are considered the main spans and consist of steel trusses and concrete decks. The other three spans are considered approach spans and consist of plate girders. The overall bridge length is 1,795 feet and has been in service since 1968, however some structural repairs were made in 1992. The average daily traffic (ADT) count was 70,787 in 1998 with a 4.5% estimated traffic growth during the life of the bridge. Girders span from the west abutment, continuously over pier 1 to pier 2, in the mainline and ramp spans. The floor beams rest on sole plates, which are welded to the top flange of the girder, so the girder and slab are non-composite. The mainline and ramp spans have two girders in each direction. In 1992, the east and west bound main line spans were tied together with diaphragms and floor beam splices. Span 3 consists of rolled and built-up members in each of the four, constant depth trusses. The floor beams are spaced at 11' 7" and rest on top of the girders. The trusses consist of welded plates or rolled I sections and there are no stringers in span 3. Spans 7, 8, and 9 consist of rolled and built-up members in each of the four, constant depth trusses. The trusses are welded into box shapes. The tension members have welded intermediate stay plates and the compression members have welded perforated plates. Figure 1. Patroon Island Bridge. Figure 2. Location of the Patroon Island Bridge. 2 Spans 4, 5, and 6 consist of built up truss members. The compression members consist of two main plates and perforated cover plates. The tension truss members consist of main plates with intermediate stay plates and diaphragms. During construction in 1968, the intermediate stay plates and diaphragms were connected to the inside of the truss plates with fillet welds. In 1992, some of the intermediate stay plates and diaphragms were removed and new plates were reconnected with bolts to increase the fatigue strength. Figure 3 shows a bolted intermediate stay plate. The cantilever and interior floor beam webs in spans 4, 5 and 6 are connected to the truss node gusset plates using steel angles, but the top flanges are made continuous with a top strap plate that is not connected to the truss (See Figures 4, 5 and 6). Many cracks have been observed in the upper portion of the floor beam web, near the top strap plate. This is a critical connection, but the fatigue life for this detail could not be determined because cracks exist. Figure 3. Bolted intermediate stay plate. Figure 4. Floor beam to truss connection. 3 Span 10 originally had independent spans to facilitate the east and west bound lanes. In 1992 the east and west bound spans were tied together with diaphragms, floor beam splices and a closure pour. Span 10 has bolted sole plates on the top flange to act as a spacer for the floor beams. The stringer webs are bolted to the web of the floor beams to allow shear transfer, but limit moment transfer. There are several flange plate transitions that are connected using groove welds. B. CURRENT STATUS OF THE BRIDGE Several structural components experienced fatigue cracks. The bridge and two ramp structures have a documented history of cracks in connection welds and floor beams. These include the webs of floor beams (Figures 4-6), sole plates between the floor beams and girders (Figure 9), truss to bearing welds (Figures 7-8), and transverse web stiffeners (Figure 9). Many of the recent problems have been related to the floor beam webs in spans 4, 5 and 6 (Figures 4-6). The cause of this cracking was attributed to the deformation of the truss in the longitudinal direction with respect to the slab and local stress concentrations in the upper portion of the web, near the truss. The floor beam web is attached to the truss using an angle. A top strap plate creates continuity between the top flanges of the floor beam on each side of the truss, but is not attached to the truss. The truss is exerting an out of plane load on the web of the floor beam. The floor beams are constrained by the stringers which are connected to the slab. The slab is very stiff along the length of the bridge, when compared to the top truss chords in the bridge, but the web of the floor beam must transmit the shear between the truss and the slab. In addition to the out of plane shear stresses in the floor beam web, there are also stresses
Details
-
File Typepdf
-
Upload Time-
-
Content LanguagesEnglish
-
Upload UserAnonymous/Not logged-in
-
File Pages61 Page
-
File Size-