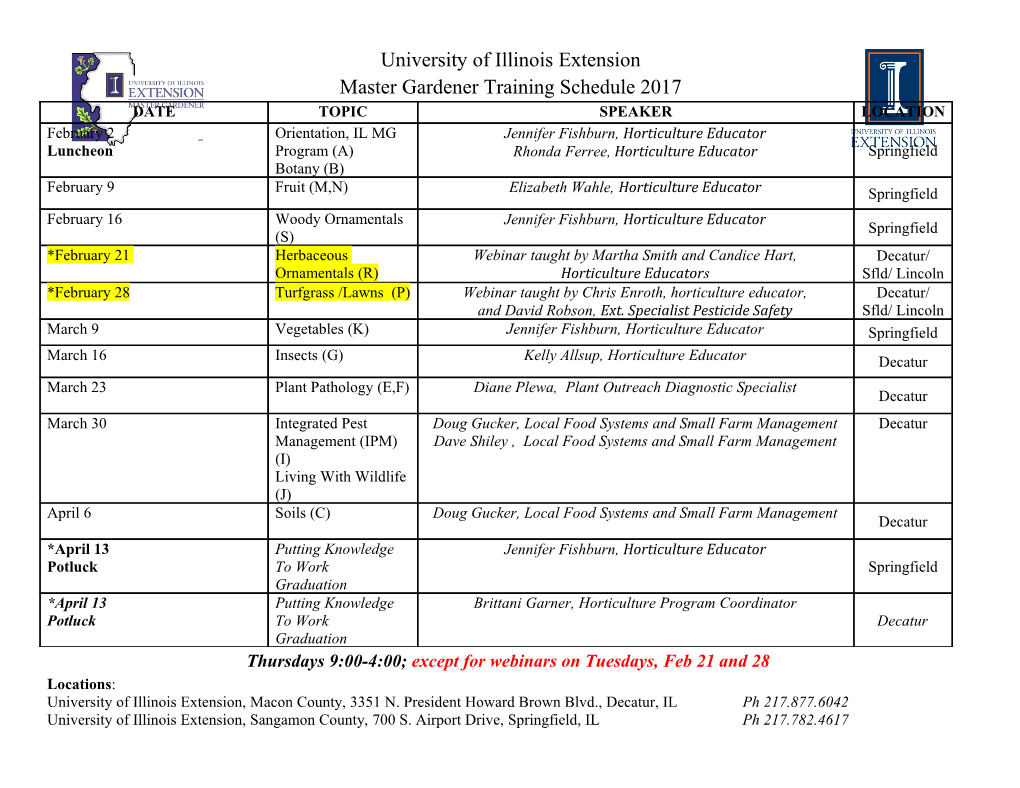
Real-time observation of ligand-induced allosteric transitions in a PDZ domain Olga Bozovica,1 , Claudio Zanobinia,1, Adnan Gulzarb,1, Brankica Jankovica, David Buhrkea , Matthias Postb , Steffen Wolfb , Gerhard Stockb,2 , and Peter Hamma,2 aDepartment of Chemistry, University of Zurich, 8057 Zurich, Switzerland; and bBomolecular Dynamics, Institute of Physics, University of Freiburg, 79104 Freiburg, Germany Edited by Martin Gruebele, University of Illinois at Urbana–Champaign, Urbana, IL, and approved August 24, 2020 (received for review June 23, 2020) While allostery is of paramount importance for protein regula- Known for their modest conformational change upon ligand- tion, the underlying dynamical process of ligand (un)binding at binding, PDZ domains are considered prime examples of one site, resulting time evolution of the protein structure, and dynamic allostery (4, 6, 19). PDZ domain-mediated interactions change of the binding affinity at a remote site are not well play a pivotal role in many signal transduction complexes (20, understood. Here the ligand-induced conformational transition in 21). Allosteric information flow in PDZ domains is thought to a widely studied model system of allostery, the PDZ2 domain, be transduced via conserved allosteric networks in the protein is investigated by transient infrared spectroscopy accompanied (4, 22–25). The system considered here is the PDZ2 domain by molecular dynamics simulations. To this end, an azobenzene- from hPTP1E (human tyrosine phosphatase 1E) and a RA-GEF- derived photoswitch is linked to a peptide ligand in a way that 2 peptide derivative (Ras/Rap1 associating guanidine nucleotide its binding affinity to the PDZ2 domain changes upon switch- exchange factor 2) (26) with an azobenzene moiety linked as pho- ing, thus initiating an allosteric transition in the PDZ2 domain toswitch (27) (Fig. 1). It was recently reported for a very similar protein. The subsequent response of the protein, covering four system that the phosphorylation of the serine (−2) residue, a decades of time, ranging from ∼1 ns to ∼10 µs, can be ratio- common target in regulatory processes of PDZ domains (28), nalized by a remodeling of its rugged free-energy landscape, leads to an approximately five- to sevenfold difference in the with very subtle shifts in the populations of a small number affinity toward the PDZ2 domain (29). We will see that the bind- of structurally well-defined states. It is proposed that struc- ing affinity can be perturbed to the same extent (approximately turally and dynamically driven allostery, often discussed as lim- fivefold) by introducing such a photoswitchable element on the BIOPHYSICS AND COMPUTATIONAL BIOLOGY iting scenarios of allosteric communication, actually go hand-in- ligand instead. Since the PDZ2 domain is not modified at all, this hand, allowing the protein to adapt its free-energy landscape to strategy leads to a much less artificial construct than obtained in incoming signals. our previous study (30, 31), where the photoswitch was covalently linked across the binding pocket of the PDZ2 domain. In addi- allostery j transient infrared spectroscopy j molecular dynamics tion, using the ligand as a trigger, one can apply this strategy to simulations j PDZ domains virtually any system. By photoisomerizing the azobenzene moiety, we change the llostery represents the coupling of two sites in a protein or binding affinity of the ligand at a precisely defined point in time. Aa protein complex, where the binding of a ligand to the dis- We employ time-resolved vibrational spectroscopy in connection tal site modifies the affinity at the active site (1). Since biological with an isotope labeling strategy to monitor the structural change function is intimately related to protein structure, ligand-induced of the protein in real time and perform extensive (more than changes of the protein’s function (e.g., the transition from an 0.5 ms aggregate simulation time) all-atom nonequilibrium MD inactive to an active state) are often associated with a change of the protein’s mean structure (2). On the other hand, ligand Significance (un)binding may also alter the protein’s flexibility, which changes the variance of the structure and gives an entropic contribution Allostery is a process in which a signal sensed upon ligand- to the free energy (3). Referring to the associated change of binding at a distal site is transduced to the effector site, the structural fluctuations, the latter scenario, termed “dynamic allowing for regulation of the activity of the latter. The prop- allostery,” has been invoked to explain apparent absence of agation of an allosteric signal is a nonequilibrium process, conformational change upon ligand (un)binding (3–10). Study- but neither the nature of the signal is known on a molecular ing the effects of dynamic allostery has been mainly done by level (e.g., whether it is structural or dynamical properties that NMR spectroscopy (11–13), which, however, only accounts for change) nor its speed. The real-time observation of such a sig- equilibrium dynamics. nal requires the design of protein systems, in which one can While both models, structural change vs. dynamic change, synchronize ligand (un)binding events. Such a design is pre- may appear plausible, the nature of the “allosteric signal” is sented here, allowing us to investigate its allosteric transition not known. A stringent examination ultimately requires us to in unprecedented detail. study the genesis of allostery. This includes three steps: 1) the (un)binding of a ligand (usually initiated by a change of its con- Author contributions: O.B., S.W., G.S., and P.H. designed research; O.B., C.Z., A.G., B.J., centration) (14) causes 2) the atoms of the protein to undergo D.B., and M.P. performed research; O.B., C.Z., A.G., B.J., M.P., S.W., G.S., and P.H. analyzed a nonequilibrium time evolution, which 3) eventually leads to data; and O.B., C.Z., S.W., G.S., and P.H. wrote the paper.y a change of the binding affinity at a remote site of the protein. The authors declare no competing interest.y This so-called “allosteric transition” is a nonequilibrium process This article is a PNAS Direct Submission.y and has been observed directly only rarely, in part, because the Published under the PNAS license.y smallness of the structural changes makes the transition path- 1 O.B., C.Z., and A.G. contributed equally to this work.y ways challenging to observe experimentally (15) and also because 2 To whom correspondence may be addressed. Email: [email protected] or of the time-scale limitations of molecular dynamics (MD) simu- [email protected] lations (16–18). In this work, we outline an approach to study This article contains supporting information online at https://www.pnas.org/lookup/suppl/ the first two steps, i.e., the ligand-induced allosteric transition, doi:10.1073/pnas.2012999117/-/DCSupplemental.y employing a PDZ2 domain as model system. First published October 5, 2020. www.pnas.org/cgi/doi/10.1073/pnas.2012999117 PNAS j October 20, 2020 j vol. 117 j no. 42 j 26031–26039 Downloaded by guest on September 28, 2021 iment. Nevertheless, we will be able to observe the adaptation of the protein to a perturbed peptide conformation in the bind- ing pocket and its transition to unspecific binding on the protein surface. We investigate the ligand-induced conformational transition with the help of transient IR spectroscopy in the range of the amide I band (see Materials and Methods for details) (42–44). This band originates from mostly the C=O stretch vibration of the peptide/protein backbone and is known to be strongly structure-dependent (45). While one cannot invert the prob- lem and determine the structure of a protein from the amide I band, any change in protein structure will cause small but distinct changes in this band (Fig. 2 A–C). Fig. 1. Ligand-switched PDZ2 domain. Main secondary structural elements Fig. 2 shows the transient IR response in the spectral region and Cα distances d20,71 and d4,55 discussed below (Results, MD Simulations) of the amide I vibration after photoswitching in either the are indicated. In the trans conformation of the photoswitch (red), the lig- trans-to-cis (Fig. 2D–F) or the cis-to-trans direction (Fig. 2 G– and (blue) fits well in the binding pocket, while it starts to move out when I). To be directly comparable, the two datasets were scaled switching to . cis in a way that they refer to the same amount of isomeriz- ing molecules and not the same amount of excited molecules. The scaling took into account the different pump-pulse ener- simulations combined with Markov modeling to interpret the gies used in the experiments (Materials and Methods), cross- experimental results in terms of the structural evolution of the sections (23,500 cm−1M−1 for trans at 380 nm vs. 2,000 system. We find that the mean structural change of the protein is cm−1M−1 for cis at 420 nm) (27), and isomerization quantum rather small. However, in both experiment and MD simulations, yields (8% for trans-to-cis switching and 62% for cis-to-trans the free-energy surface of the protein can be characterized by switching) (46). a small number of metastable conformational states. In agree- Selective isotope labeling can be used to disentangle the ment with the view of allostery as an interconversion between contribution of the peptide ligand from that of the protein. the relative population of metastable states, we see how the 13C15N-labeling of the protein backbone down-shifts the vibra- ligand-induced response of the PDZ2 domain is best described tional frequency of the amide I band by ≈25 cm−1.
Details
-
File Typepdf
-
Upload Time-
-
Content LanguagesEnglish
-
Upload UserAnonymous/Not logged-in
-
File Pages9 Page
-
File Size-