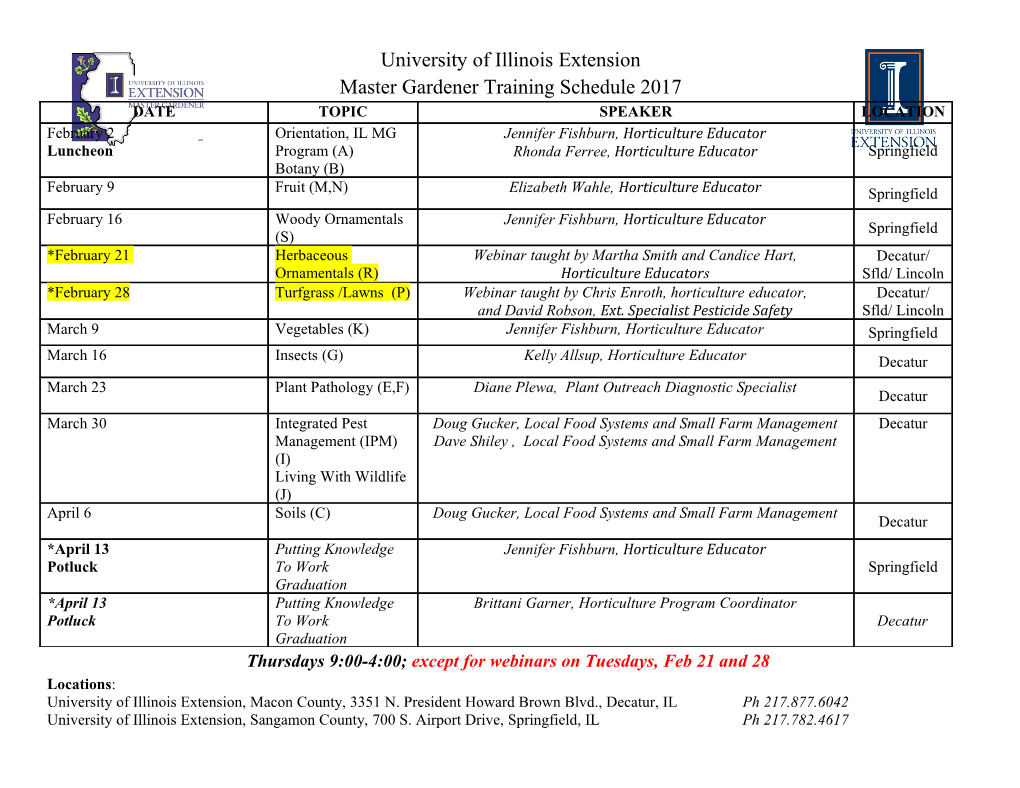
Impacts of soil electrical conductivity and compost mulches on phylloxera establishment and abundance Kevin Powell, Ginger Korosi, Bernadette Carmody and Rebecca Bruce Department of Primary Industries, Rutherglen Centre, Biosciences Research Division, RMB 1145 Chiltern Valley Road, Rutherglen, Victoria 3685, Australia Corresponding author: [email protected] Introduction Grape phylloxera ( Daktulosphaira vitifoliae Fitch) is present in most grape-growing countries and can attack both the roots and foliage of grapevines. The root-galling form of phylloxera causes significant economic damage to Vitis vinifera L. and can also survive on the roots of ‘resistant’ rootstocks. In Australia grape phylloxera is a quarantine pest and is restricted to phylloxera infested zones in Victoria and New South Wales. Eighty-three genotypic clones of phylloxera have so far been identified in Australia (Umina et al. 2007) and some strains predominate in the different geographical regions. The degree of damage to the grapevine root system and the risk of phylloxera establishment through quarantine breakdown is predominantly influenced by the virulence of the phylloxera genetic strain (Powell 2008, 2011). Long-term management of root-galling strains of phylloxera has predominantly focused on recommending resistant rootstocks and developing robust quarantine protocols. This focus has largely ignored the impact that the soil environment, which is after all phylloxeras’ predominant habitat, has on the insect-host plant interactions. Future phylloxera management needs to consider a more integrated approach and where the influence of soil physiochemical properties on phylloxera establishment, subsequent abundance and the risk of spread are recognised. Newly developed options for targeted early detection of phylloxera are being developed, where soil factors are considered, include the use of a soil molecular DNA probe, trapping techniques and the use of soil electromagnetic induction surveys. Soil chemistry can also influence phylloxera establishment and abundance (Powell et al. 2003; Reisenzein et al. 2007) and their may be potential to modify these soil-pest interactions through soil management. Alternative approaches to phylloxera management using organic mulches may also be a potential option particularly when low virulent genetic strains of phylloxera are detected. 1 Phylloxera genetics and rootstock selection Different phylloxera strains can establish and develop colonies on resistant rootstocks to varying levels of adaptability and resistance-breaking biotypes have been reported on rootstock hybrids with partial V. vinifera parentage (Granett et al. 1985). However the genetic variability within phylloxera populations has been poorly understood until relatively recently. The development of improved molecular techniques over the last decade has seen an increased understanding and clarification of the life-cycle and genetics of grapevine phylloxera. Firstly with the development of random amplification of polymorphic DNA (RAPD) and amplified fragment length polymorphism (AFLP) genetic markers (Fong et al. 1995; Forneck et al. 2000), and more recently with nuclear mitochondrial DNA markers (Corrie et al. 2003; Vorwerk and Forneck 2006). The complexity and range of genetic diversity characterised using these techniques has highlighted the potential for breakdown in phylloxera-resistant rootstocks and the need for selection of rootstocks based on phylloxera and grapevine genotypic background to reduce the risk of phylloxera transfer on machinery, grape products or footwear (Deretic et al. 2003; Dunstone et al. 2003; Korosi et al. 2009). The use of nuclear DNA microsatellite markers has resulted in the characterisation and geographical distribution of over 80 distinct phylloxera genotypes in Australia (Umina et al. 2007). Of these known genotypes most are root-feeding (radicicolae) only, but others are purely leaf-galling (gallicicole) and relatively few appear to be able to combine both a radicicolae and gallicicole life habitat (Corrie et al. 2003, 2004). Differing virulence levels of root-galling genetic strains on commercially-available rootstocks (Figure 1), novel hybirds and ungrafted V. vinifera have been reported (Korosi et al. 2007; Korosi et al. 2011a, b). This has important implications for management of ungrafted vines and selection of phylloxera- resistant rootstocks. In Australia when a new outbreak of phylloxera is detected insects are now routinely DNA typed and their population dynamics monitored in the field (Powell et al. 2003; Herbert et al. 2006). This assists the grower in determining both the choice of rootstock and timelines for a replanting program based on the insect genetics and population biology. DNA typing can also aid in traceback procedures to determine the origin of an infestation (Umina et al. 2007). 2 G1 Phylloxera survival 1 5BB Kober Ramsey 0.9 Schwarzmann Shiraz (control) 0.8 0.7 0.6 0.5 0.4 0.3 0.2 Kaplan-Meier estimate Kaplan-Meier 0.1 0 0 1 2 3 4 5 6 7 8 weeks G19 phylloxera survival 1 5BB Kober Ramsey 0.9 Schwarzmann Shiraz (control) 0.8 0.7 0.6 0.5 0.4 0.3 0.2 Kaplan-Meier Estimate Kaplan-Meier 0.1 0 0 1 2 3 4 5 6 7 8 weeks Figure 1. Estimate of relative survival of two grapevine phylloxera genetic strains (a) G1 and (b) G19 on four rootstocks tested using an excised root bioassay system. Quarantine and detection Grapevine phylloxera is a significant quarantine pest in Australia since its first detection in 1877 near Geelong, Victoria (Powell 2008). Quarantine protocols and regulations relating to the movement of grape material and vineyard machinery (NVHSC 2009) have been somewhat successful in minimising the risk of widespread distribution of phylloxera throughout all states in Australia. However, over the last decade a number of new infestations have been confirmed within the state of Victoria. The most recently created Phylloxera Infested Zone (PIZ) is the Maroondah PIZ, located in the Yarra Valley. Phylloxera was first detected there in December 2006, and at that time was thought to be contained to a single 3 vineyard until 2008-2010 when it was detected in a further 4 vineyards within the region resulting in an extension of the quarantine zone. This reaffirmed an important issue surrounding phylloxera detection; i.e. that an infestation may become established within a vineyard several years prior to physical visual evidence of vine stress. Early detection techniques are vital to contain the spread of phylloxera particularly highly virulent strains, such as G1 and G4 which survive and reproduce extremely well on ungrafted V. vinifera (Herbert et al. 2010). Some low virulence phylloxera genetic strains are also important and can be relatively difficult to detect because they cause less root damage and hence visual symptoms above ground are less apparent. Early detection of all phylloxera strains is essential to ensure the sustainability and profitability of the viticulture industry in Australia. Late detections can result in insignificant economic cost to affected growers, with replanting onto phylloxera resistant rootstocks costing up to AU$25,000 per hectare and the additional costs associated with implementation of quarantine protocols. Conventional detection The first stage in any phylloxera management program is to detect the insect, which is a challenge due to its small size and seemingly random spatial distribution. A range of detection options have been developed (Renzullo et al. 2004; Herbert et al. 2007; Powell 2007a). The root-galling form spends most of its life below-ground phylloxera feeding on the grapevine root system, although in spring and summer it does emerge from onto the soil surface causing increased quarantine risks (Powell et al. 2000). It can be present in high abundance or in relatively low numbers depending on its genetic identity, its host plant genotype, climatic conditions and the soil environment. Generally the first indication that phylloxera may be present in a vineyard is shown by show stress symptoms in the foliage or canopy. This can be expressed as premature senescence in autumn, stunting of lateral shoot growth, reduced grape yields, reduced overall vigour or a general weak spot within a group of vines. However, once a weak spot is detected phylloxera has been present for several years and is highly likely to have spread to other vines which appear seemingly vigorous and asymptomatic. Conventional detection methods rely on systematic vine root surveys to inspect for the physical presence of phylloxera, but this technique is costly due its labour intensive nature. Remote sensing systems to generate multispectral imagery for identification of areas of reduced photosynthetically-active biomass on both a vineyard- and area-wide scale (Renzullo et al. 2004; Frazier et al. 2004) have also been used. While remote sensing is successful in identifying areas of poor canopy vigour for follow-up ground survey, these ‘weak spots’ may be caused by any number of non-specific stresses including water or nutrient stress and unrelated pest and diseases (Renzullo et al. 2004). Healthy canopy vigour, 4 particularly in wet seasons, may also disguise the expression of above-ground signs of root degradation, particularly if the infesting phylloxera genotype is either of low virulence, relatively unsuited to the environmental conditions, or if the vineyard is planted with tolerant rootstock varieties (Korosi et al. 2007; Herbert et al. 2008). Emerging detection techniques Three
Details
-
File Typepdf
-
Upload Time-
-
Content LanguagesEnglish
-
Upload UserAnonymous/Not logged-in
-
File Pages18 Page
-
File Size-