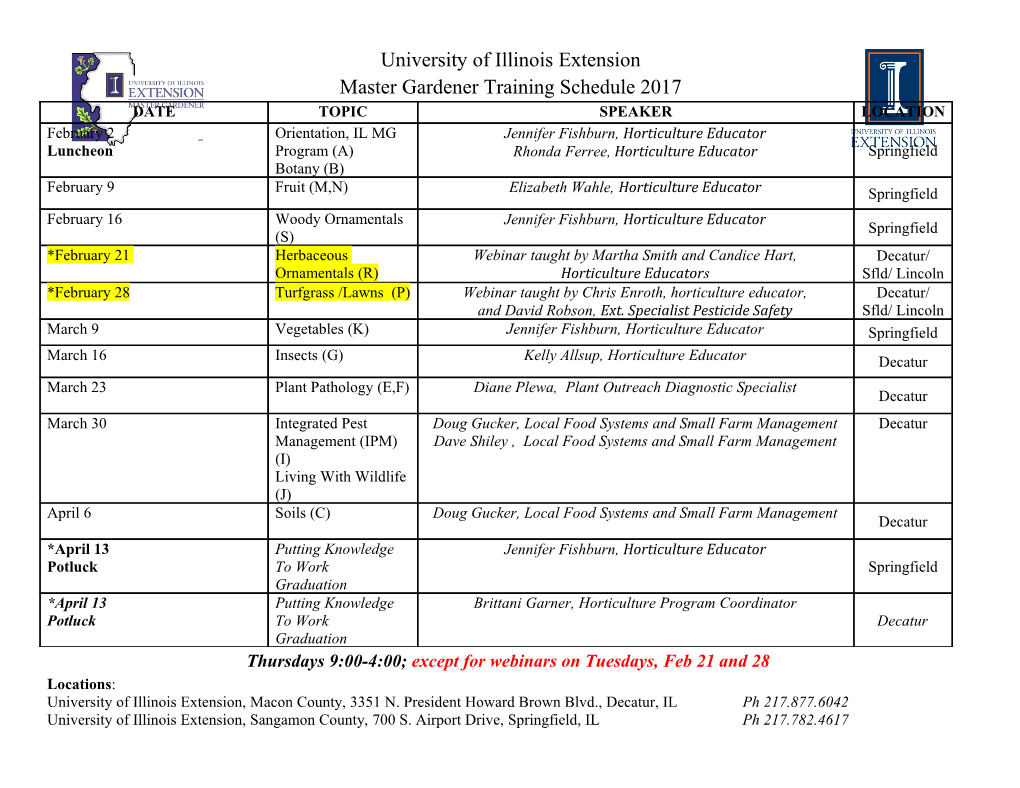
The Great Escape: Drivers of Diel Vertical Migration in Zooplankton and the Role of Dissolved Organic Carbon BIOS 35502-01: Practicum in Environmental Field Biology Joseph Nowak Advisor: Amaryllis Adey 2019 Abstract In nearly all aquatic environments, zooplankton are observed to inhabit deeper waters during the day and surface waters at night in a process known as diel vertical migration (DVM). There are several proposed theories for why this phenomenon occurs including the avoidance of UV radiation and visual predators, but not much is known about the role of dissolved organic carbon (DOC). In this study, zooplankton tows were performed on the epilimnion of six lakes, three with high DOC and three with low DOC, during the day and at night. Two zooplankton orders, copepods and cladocerans, along with the zooplankton predator Chaobrous were counted in each sample. Copepods and Chaobrous exhibited significant DVM across the six lakes, while Cladocera and Chaobrous showed greater migration in lakes with high DOC. Though the exact driver of the difference in migration patterns were not identified, theories are provided and recommendations for future studies are given. Introduction Diel vertical migration (DVM) is a tactic utilized by the majority of marine and freshwater zooplankton taxa, comprising the world’s largest animal migration with respect to biomass (Hays 2003). During the normal DVM pattern, zooplankton reside deeper in the water column during the day and spend nights at the surface. The transitions between these states occur at dusk (ascent) and dawn (descent). The current literature cites two primary drivers of DMV: ultraviolet (UV) radiation and visual predation. Sunlight contains short wavelengths of UV radiation that can cause damage to the DNA and membranes of zooplankton that remain in surface waters for extended periods of time (Rautio and Tartarotti 2010). Consequently, it is hypothesized that zooplankton migrate downwards at dawn in order to avoid potentially lethal UV rays that are present during the day. Though UV radiation can penetrate well past 10 meters in highly transparent and high-altitude lakes, the depth at which only 1% of 380-nm UV-A penetrates is less than 2 meters in most lakes (Williamson et al. 1996). As a result, many researchers claim that UV radiation can only be responsible for DVM to depths of a few meters (Lampert 1989; Williamson et al. 2011). 1 Visual predation evasion is therefore most often considered the primary driver of DVM. The underlying assumption for this theory is that herbivorous zooplankton feed on phytoplankton residing in surface waters (Williamson et al. 2011). However, visual predators such as planktivorous fish and Chaoborus, a type of midge larva, can better locate prey in these brighter waters (Williamson et al. 2011). Zooplankton thus stay in deeper, darker waters during daylight hours, coming up to feed at night when visual predation is considerably more difficult (Williamson et al. 2011). In other words, the benefit of reduced chance of mortality from predation outweighs the cost of a reduced feeding window, or as one research team aptly puts it, “better hungry than dead” (Kremer and Kremer 1988). Numerous studies have tested and confirmed the presence of this phenomenon. For example, multiple studies have found that zooplankton DVM increases in the presence of planktivorous fish or fish kairomones, while others have determined that smaller zooplankton migrate less than larger ones since they are less prone to visual predation (Dodson 1988; Van Gool E. and J. Ringelberg 2002; Bollens and Frost 1991; Thys and Hoffmann 2005). Considering the great diversity of zooplankton, it is important to note that DVM behavior is not consistent among all taxa. For instance, Daphnia and other cladocerans exhibit a stronger propensity to avoid UV radiation, while copepods have greater UV tolerance and may even seek it out (Hessen 1994; Kessler et al. 2008; Williamson et al. 2011). Unfortunately, there is a tendency in the scientific community to focus solely on Daphnia when researching DVM in lakes, especially with respect to changes in dissolved organic carbon (DOC) (Williamson et al. 2011). In lakes and other freshwater bodies, as DOC concentrations increase there can be significant effects on the structure and processes of ecosystems. DOC most often enters such 2 systems in the form of dissolved organic matter (DOM), which can enter a body of water via two types of pathways, autochthonous and allochthonous (Solomon et al. 2015). Autochthonous sources are internal, derived from primary production within the aquatic system, while allochthonous sources are external, originating from primary production outside the system (Solomon et al. 2015). As a result of climate change, the latter type of DOC inputs has increased in the majority of regions in a phenomenon known as “global browning” (Solomon et al. 2015). Consequently, understanding the response of lake ecosystems to changing DOC concentrations is critical. Due to their position among the lowest level heterotrophs in lake ecosystems, the effects of changes in DOC on zooplankton migration have important implications on higher trophic levels. In addition, DVM causes zooplankton to remove nutrients such as carbon and nitrogen from surface waters at night through feeding and release them in deeper waters during the day via respiration and excretion (Schnetzer & Steinberg 2002). Therefore, changes in DVM may have a profound effect on nutrient cycling that impacts the entire lake environment. While some studies have been conducted, there is still no consensus on how DVM differs in lakes of varying DOC concentrations. One potential effect of “global browning” is a reduction in the amount of light penetrating through a given lake and a subsequent decrease in total primary productivity (Solomon et al. 2015). Though a reduction in phytoplankton would likely decrease zooplankton populations, it is unclear how this would impact DVM. In less transparent lakes, phytoplankton are also more constrained to surface waters, which may increase DVM as long as visual predators are present (Moeller 1994). However, some studies have found that chlorophyll 3 concentrations, which serve as a proxy for herbivore food availability, are higher in deeper waters than near the surface (Kiefer et al. 1972; Fee 1976). As for predation, more transparent lakes in general have lower fish densities (Downing et al. 1990). In less transparent lakes, on the other hand, turbidity decreases the maximum distance at which fish can identify prey (Sweka and Hartman 2003). Both of these factors are believed to favor an increase in DVM in less transparent (high DOC) lakes due to the increased risk of predation and greater concealment benefit gained from going deeper in the water column. The effect of DOC on UV radiation is less clear. Some researchers theorize that DOM shields zooplankton from harmful UV radiation, while recent studies have disputed this assumption, arguing that UV radiation reacts with DOM to produce reactive oxygen species (ROS) capable of damaging zooplankton DNA (Solomon 2017). It is therefore difficult to determine if DOC changes the effect of UV radiation on DVM. While there are lingering questions about the influence of DOC on DVM, even less is known about these effects with respect to different zooplankton taxa. In this study, zooplankton were collected during the day and night from six lakes, three with high DOC and three with low DOC, to determine if DVM differed between copepods and cladocerans. Chaoborus density was also measured at day and night for each lake to establish if a link exists between predation and DVM. I hypothesized that both copepods and cladocerans would both exhibit normal DVM in all lakes, but that migration would not be as detectable in low DOC lakes. In addition, I predicted that DVM would also be found in Chaoborus and that it would also be less apparent in low DOC lakes since they are also consumed by fish and may follow the zooplankton migratory pattern in order to feed. 4 Methods All sampling and analysis were conducted at the University of Notre Dame Environmental Research Center (UNDERC). The three lakes chosen for the low-DOC group were Bay (5.99 mg/L), Crampton (5.49 mg/L), and West Long (8.09 mg/L), while those selected for the high-DOC group were Hummingbird (25.9 mg/L), Morris (17.49 mg/L, and Tenderfoot (12.88 mg/L). DOC data for these lakes were taken from previous DOC records provided by Kelly et al. (2014), Craig et al.(2015), and Weidel et al (2017). For each lake, all measurements were conducted at the deepest part of each lake based on bathymetric maps provided by UNDERC. For lakes with multiple deepest areas, the one closest to the dock was chosen for convenience (Figure 1). For each lake, a temperature vs depth curve plot was created for the first 4 meters in order to determine the depth of the epilimnion before sampling (Figure 2). Four zooplankton samples per lake were collected via a 12-inch diameter zooplankton tow, two during the day and two at night. These tows were lowered to the depth marking the end of the epilimnion and were raised to ensure as much verticality as possible. Daytime samples were conducted between 11am and 1pm, while nighttime samples were performed between 11pm and 1am. All samples were taken during the same week and in similar weather conditions (clear to partly cloudy, no precipitation). These samples were filtered using 35 m mesh, and the zooplankton were preserved in pee cups with ethanol. For counting, samples were removed from ethanol and mixed with deionized water. For each sample, the mass of the empty cup and the cup with sample were recorded. The sample was then stirred, and a subsample was taken using a pipette and placed onto a petri dish with grid.
Details
-
File Typepdf
-
Upload Time-
-
Content LanguagesEnglish
-
Upload UserAnonymous/Not logged-in
-
File Pages21 Page
-
File Size-