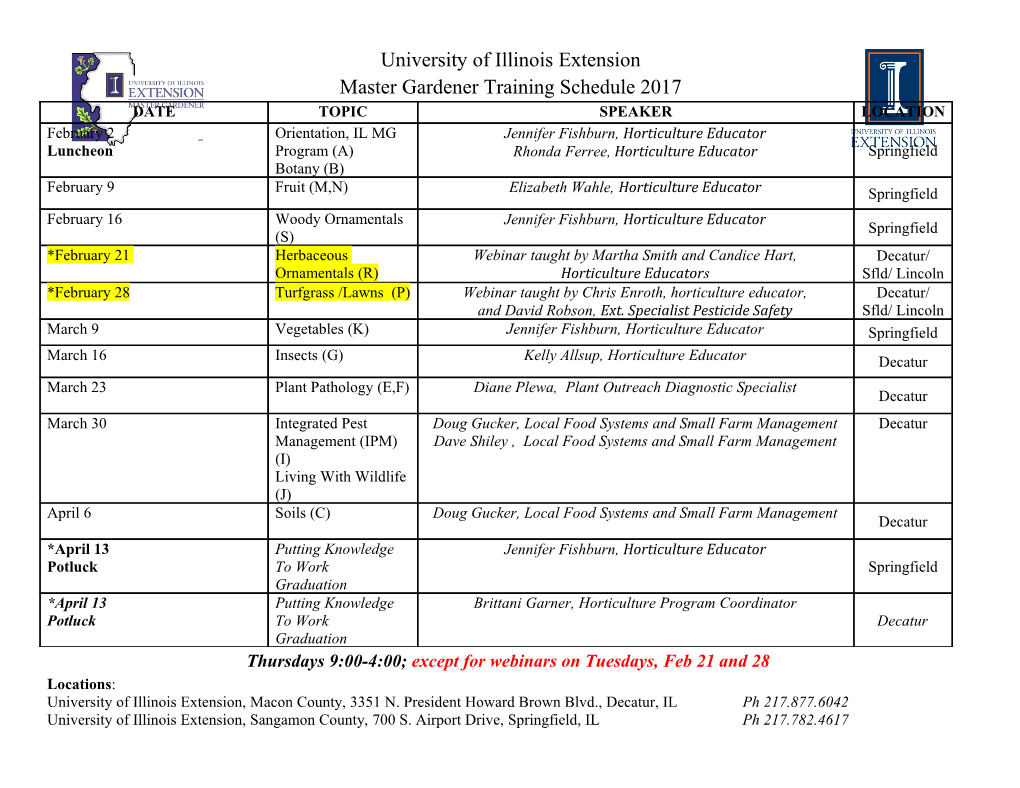
1 Disruption of Thalamic Functional Connectivity is a Neural Correlate of 2 Dexmedetomidine-Induced Unconsciousness 3 Oluwaseun Akeju1,†,*, Marco L. Loggia2,3,†, Ciprian Catana2, Kara J. Pavone1, Rafael Vazquez1, 4 James Rhee1, Violeta Contreras Ramirez1, Daniel B. Chonde2, David Izquierdo-Garcia2, Grae 5 Arabasz2, Shirley Hsu2, Kathleen Habeeb4, Jacob M. Hooker2, Vitaly Napadow2,3, Emery N. 6 Brown1,5,6, Patrick L. Purdon1,6, * 7 8 1Department of Anesthesia, Critical Care and Pain Medicine, Massachusetts General Hospital, 9 Harvard Medical School, Boston, MA 10 2MGH/MIT/HMS Athinoula A. Martinos Center for Biomedical Imaging, Charlestown, MA 11 3Department of Anesthesiology, Perioperative and Pain Medicine, Brigham and Women’s 12 Hospital, Harvard Medical School, Boston, MA 13 4Clinical Research Center, Massachusetts General Hospital, Boston, MA 14 5Department of Brain and Cognitive Science, and Institute for Medical Engineering and 15 Sciences, Massachusetts Institute of Technology, Cambridge, MA 16 6Harvard-Massachusetts Institute of Technology Division of Health Sciences and Technology, 17 Massachusetts Institute of Technology, Cambridge, MA 18 19 †These authors contributed equally 20 * To whom correspondence should be addressed: 21 [email protected] (O.A) 22 [email protected] (P.L.P.) 23 24 25 One Sentence Summary: Thalamo-cortical functional connectivity patterns are correlated with 26 unconsciousness and recovery. 2 27 Abstract 28 Understanding the neural basis of consciousness is fundamental to neuroscience 29 research. Disruptions in cortico-cortical connectivity have been suggested as a primary 30 mechanism of unconsciousness. By using a novel combination of positron emission tomography 31 and functional magnetic resonance imaging, we studied anesthesia-induced unconsciousness 32 and recovery using the α2-agonist dexmedetomidine. During unconsciousness, cerebral 33 metabolic rate of glucose and cerebral blood flow were preferentially decreased in the thalamus, 34 the Default Mode Network (DMN), and the bilateral Frontoparietal Networks (FPNs). Cortico- 35 cortical functional connectivity within the DMN and FPNs was preserved. However, DMN 36 thalamo-cortical functional connectivity was disrupted. Recovery from this state was associated 37 with sustained reduction in cerebral blood flow, and restored DMN thalamo-cortical functional 38 connectivity. We report that loss of thalamo-cortical functional connectivity is sufficient to 39 produce unconsciousness. 2 3 40 Introduction 41 Understanding the neural mechanisms of consciousness is a fundamental challenge of 42 current neuroscience research. We note that precise definitions of the words “consciousness” 43 and “unconsciousness” remain ambiguous. However, loss of voluntary response in a task is an 44 effective and clinically relevant definition of unconsciousness. Over the past decade, important 45 insights have been gained by using functional magnetic resonance imaging (fMRI) to 46 characterize the brain during sleep, and disorders of consciousness (DOC) such as coma, 47 vegetative states and minimally conscious states (Boly et al., 2009; Cauda et al., 2009; 48 Fernandez-Espejo et al., 2012; Greicius et al., 2008; Horovitz et al., 2009; Horovitz et al., 2008; 49 Larson-Prior et al., 2009; Picchioni et al., 2014; Samann et al., 2011; Vanhaudenhuyse et al., 50 2010). In addition to being critical for patients to safely and humanely undergo traumatic 51 surgical or diagnostic procedures, anesthesia has long been recognized as a tool for studying 52 the neural mechanisms of loss and recovery of consciousness (Beecher, 1947). 53 54 Disruption of cortico-cortical connectivity has been proposed as a central mechanism to 55 explain unconsciousness associated with general anesthesia, sleep and DOC (Alkire et al., 56 2008; Boly et al., 2009; Boveroux et al., 2010; Cauda et al., 2009; Jordan et al., 2013; Langsjo 57 et al., 2012; Mashour, 2006; Massimini et al., 2005; Vanhaudenhuyse et al., 2010). However, 58 recent evidence suggests that cortico-cortical functional connectivity may be maintained during 59 unconsciousness (Greicius et al., 2008; Horovitz et al., 2008; Larson-Prior et al., 2009). To 60 develop more precise neuroanatomic and neurophysiological characterizations of this and other 61 putative mechanisms, studies of anesthetic-induced unconsciousness could benefit from the 62 use of multimodal imaging approaches, combined a with neurophysiological understanding of 63 the brain state induced by the anesthetic drug being studied. 64 3 4 65 A challenge in using anesthetics to study unconsciousness is stating precise, possible 66 mechanisms of anesthetic induced brain states, and using an anesthetic which acts at single 67 rather than multiple brain targets to test those mechanism (Brown et al., 2011; Franks, 2008; 68 Rudolph and Antkowiak, 2004). Among the several anesthetics in current use today, 69 dexmedetomidine is an appealing choice for testing a specific mechanism of how an anesthetic 70 alters the level of consciousness. Dexmedetomidine selectively targets pre-synaptic α2- 71 adrenergic receptors on neurons projecting from the locus ceruleus to the pre-optic area (Chiu 72 et al., 1995; Correa-Sales et al., 1992; Mizobe et al., 1996). This leads to activation of inhibitory 73 outputs to the major arousal centers in the midbrain, pons and hypothalamus producing a 74 neurophysiological and behavioral state that closely resembles NREM II sleep (Figure 1) (Akeju 75 et al., 2014; Huupponen et al., 2008; Nelson et al., 2003). Dexmedetomidine also acts at the 76 locus ceruleus projections to the intralaminar nucleus of the thalamus, the basal forebrain and 77 the cortex (Espana and Berridge, 2006). 78 79 Therefore, the aim of this study was to use a novel integrated positron emission 80 tomography and magnetic resonance imaging (PET/MR) approach characterize brain resting- 81 state network activity and metabolism during dexmedetomidine-induced unconsciousness. This 82 strategy offers several appealing features. First, PET scanning using fludeoxyglucose (18F-FDG) 83 provides a sensitive estimate of the brain’s metabolic state in terms of the cerebral metabolic 84 rate of glucose (CMRglc). Second, comparison of CMRglc results with regional cerebral blood flow 85 (rCBF) estimates obtained from pulsed Arterial Spin Labeling (pASL) fMRI signals allows a 86 direct assessment of whether, as previously reported, flow-metabolism coupling is maintained 87 during dexmedetomidine-induced unconsciousness (Drummond et al., 2008). Third, we use the 88 CMRglc results to inform the brain functional connectivity analysis derived from the Blood 89 Oxygen Level Dependent (BOLD) fMRI signals. 4 5 90 Results 91 Decreased CMRglc in the Default Mode Network (DMN), Fronto Parietal Networks (FPNs), 92 and thalamus during dexmedetomidine-induced unconsciousness 93 In 10 healthy volunteers, we used EEG recordings, and an auditory task to confirm that 94 dexmedetomidine induced a loss of voluntary responsiveness, and exhibited a 95 neurophysiological profile that was similar to non rapid eye movement (NREM) II sleep (Akeju et 96 al., 2014). We then studied 18F-FDG uptake during baseline and dexmedetomidine-induced 97 unconsciousness in these 10 healthy volunteers in a separate experiment where we defined 98 loss of consciousness as the onset of sustained eye closure and lack of response to a verbal 99 request to open the eyes (Figure 2A). During both the baseline and dexmedetomidine-induced 100 unconsciousness 18F-FDG study visits (two per subject), fMRI data were also recorded. 101 Consistent with a global decrease in glucose metabolism, we found that during the 102 unconsciousness state, there was a broad reduction in 18F-FDG standardized uptake values in 103 the brain (Figure 2- figure supplement 1A). To quantify this result, we calculated CMRglc during 18 104 the two F-FDG visits (Figure 2- figure supplement 1B). We found reduced CMRglc in thalamic, 105 frontal and parietal brain regions during the unconscious state (Figure 2B, Table 1). The 106 difference in CMRglc exhibited a spatial distribution consistent with the previously described 107 DMN (Table 1) (Damoiseaux et al., 2006; Raichle et al., 2001) and both left and right FPNs 108 (Table 1) (Damoiseaux et al., 2006; Vincent et al., 2008). We found these network specific 109 CMRglc changes interesting because the DMN has been linked to stimulus-independent thought, 110 and self-consciousness (Mason et al., 2007; Raichle et al., 2001; Vincent et al., 2008), while the 111 FPNs have been linked to conscious perception of the external environment and executive 112 control initiation (Boly et al., 2008; Vincent et al., 2008). 113 Decreased rCBF in DMN, FPN, and thalamus during dexmedetomidine-induced 114 unconsciousness 5 6 115 To further understand the effects of dexmedetomidine-induced unconsciousness on 116 rCBF and brain functional connectivity in these networks, we studied an additional 7 volunteers 117 using fMRI only (Figure 2C). Analysis of the rCBF difference maps between the unconscious 118 and baseline states, showed decreases in rCBF during unconsciousness that overlapped with 119 the same DMN and FPN regions that demonstrated reductions in CMRglc (Figure 2D, Figure 2- 120 figure supplement 1, Table 2). This is consistent with maintenance of blood flow and 121 metabolism coupling during dexmedetomidine-induced unconsciousness. 122 Decreased thalamo-cortical and cortico-cerebellar functional connectivity
Details
-
File Typepdf
-
Upload Time-
-
Content LanguagesEnglish
-
Upload UserAnonymous/Not logged-in
-
File Pages49 Page
-
File Size-