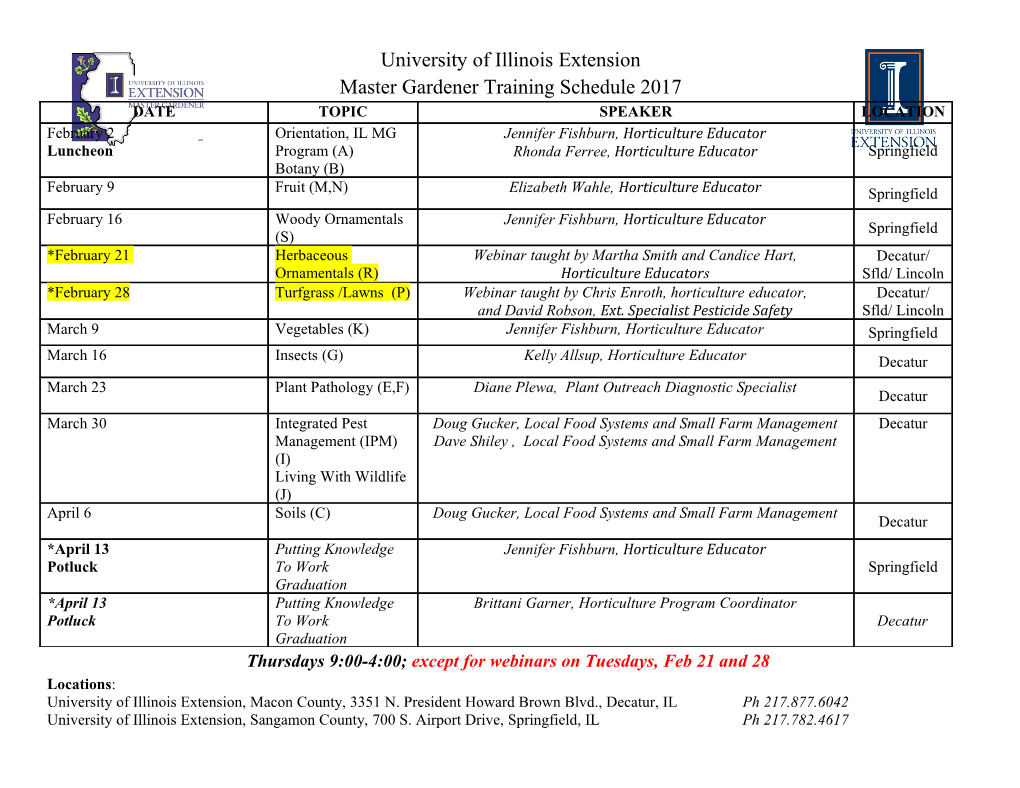
Arylsulfatase G inactivation causes loss of heparan sulfate 3-O-sulfatase activity and mucopolysaccharidosis in mice Björn Kowalewskia, William C. Lamannab,1, Roger Lawrenceb,1, Markus Dammea,1, Stijn Stroobantsc, Michael Padvad, Ina Kalusa, Marc-André Fresea, Torben Lübkea, Renate Lüllmann-Rauche, Rudi D’Hoogec, Jeffrey D. Eskob, and Thomas Dierksa,2 aBiochemistry I, Department of Chemistry, Bielefeld University, 33615 Bielefeld, Germany; bDepartment of Cellular and Molecular Medicine, Glycobiology Research and Training Center, University of California at San Diego, La Jolla, CA 92093; cLaboratory of Biological Psychology, Department of Psychology, University of Leuven, 3000 Leuven, Belgium; dBiochemistry II, Georg-August University Göttingen, 37073 Göttingen, Germany; and eDepartment of Anatomy, Christian-Albrechts-University Kiel, 24098 Kiel, Germany Edited by Stuart A. Kornfeld, Washington University School of Medicine, St. Louis, MO, and approved May 7, 2012 (received for review February 6, 2012) Deficiency of glycosaminoglycan (GAG) degradation causes a sub- Arylsulfatase G (ARSG), a recently characterized lysosomal sul- class of lysosomal storage disorders called mucopolysaccharidoses fatase with unknown physiological substrates (9), has been reported (MPSs), many of which present with severe neuropathology. Critical previously to be associated with an adult form of ceroid lipofuscinosis steps in the degradation of the GAG heparan sulfate remain enig- in dogs (10). In this study, we generated an ARSG-deficient mouse matic. Here we show that the lysosomal arylsulfatase G (ARSG) is model by targeted disruption of the Arsg gene.Inadditiontoheparan the long-sought glucosamine-3-O-sulfatase required to complete sulfate storage, we observed pathological changes common to MPS- fi the degradation of heparan sulfate. Arsg-deficient mice accumulate type LSDs and identi ed the physiological substrate of ARSG as heparan sulfate in visceral organs and the central nervous system the N-sulfoglucosamine-3-O-sulfate moiety of heparan sulfate. Our fi and develop neuronal cell death and behavioral deficits. This accu- ndings clearly demonstrate that ARSG is the long-sought glucos- amine-3-sulfatase and that its deficiency results in a unique type of mulated heparan sulfate exhibits unique nonreducing end struc- fi tures with terminal N-sulfoglucosamine-3-O-sulfate residues, MPS, which we propose to designate as MPS IIIE (San lippo E). allowing diagnosis of the disorder. Recombinant human ARSG is Results able to cleave 3-O-sulfate groups from these residues as well as fi from an authentic 3-O-sulfated N-sulfoglucosamine standard. Our ARSG-De cient Mice Show Lysosomal Storage and Cellular Alterations results demonstrate the key role of ARSG in heparan sulfate deg- in the CNS. To determine the physiological function of ARSG, we fi inactivated the gene in mice by insertion of a neomycin-resistance radation and strongly suggest that ARSG de ciency represents a fi unique, as yet unknown form of MPS, which we term MPS IIIE. cassette into exon 2 (Fig. S1A). Arsg inactivation was con rmed by Southern blot analysis, genomic PCR, and RT-PCR (Fig. S1 B– D). Arsg-knockout mice exhibited normal development, showed no lysosomes | sulfatases | Sanfilippo syndrome | mouse model obvious changes in appearance, and were fertile. Macroscopic in- spection of organs was unremarkable. In the CNS, no apparent ysosomal storage disorders (LSDs) comprise a group of atrophy was evident up to the age of 12 mo; however, several inherited metabolic diseases characterized by the accumula- neuronal and microglia populations revealed obvious vacuoles with L fl tion of storage material re ecting a defect of a lysosomal hy- prominent lysosomal storage pathology in Purkinje cells of the fi drolase or associated protein (1). De ciencies in six lysosomal cerebellum and also in perivascular and meningeal macrophages sulfatases lead to severe LSDs caused by accumulation of the (Fig. 1 A and B and Fig. S2 A and B). Thalamic and hippocampal corresponding sulfated substrate in the lysosome, resulting in the neurons revealed sporadic lysosomal inclusions (Fig. S2 C and D). impairment of lysosomal function as well as the initiation of – – Storage vacuoles showed positive periodic acid Schiff staining (Fig. pathological cascades outside the lysosomes (1 3). Arylsulfatase A 1C) and appeared partially empty after Epon embedding, indi- (ARSA) cleaves sulfated galactosylceramides (4), which, when cating water-soluble carbohydrate storage material. Transmission absent, leads to metachromatic leukodystrophy (5), whereas the fi electron microscopy (TEM) of the storage material in Purkinje other ve characterized lysosomal sulfatases [arylsulfatase B, ga- cells showed lamellar to finely granular structures (Fig. 1D). In N lactosamine-6-sulfatase, ( -acetyl)glucosamine-6-sulfatase, hep- older animals, storage material revealed autofluorescent proper- aran-N-sulfatase (sulfamidase), and iduronate-2-sulfatase] function ties at different excitation wavelengths (Fig. 1 E and E′ and Fig. S2 in the successive degradation of sulfated glycosaminoglycans E and E′). The autofluorescent material colocalized in ring-like (GAGs), namely heparan sulfate, chondroitin sulfate, dermatan ′ sulfate, and keratan sulfate (3). The lysosomal degradation of structures with the lysosomal marker Lamp1 (Fig. 1 F and F ). In the course of the disease, considerable loss of Purkinje cells was GAGs occurs stepwise beginning at the nonreducing end (NRE) of > ′ the sugar chain. Genetic deficiency of any of the enzymes involved observed, becoming clearly evident at 10 mo (Fig. 1 G and G ). Neuronal cell death was accompanied by strong microgliosis (Fig. 1 leads to mucopolysaccharidoses (MPSs), a disease group com- – ′ ′ prising 11 disorders with distinct molecular defects (6). H I ) and astrogliosis in the cerebellar cortex (Fig. 1 J and J ). Among the degradation routes for the different GAGs, heparan sulfate catabolism is the most complex, involving three glyco- sidases, three known sulfatases, and one acetyltransferase (6). Author contributions: M.D., I.K., M.-A.F., T.L., R.L.-R., R.D., J.D.E., and T.D. designed re- Impairment of any of these enzymes has been linked to a specific search; B.K., W.C.L., R.L., M.D., S.S., M.P., and R.L.-R. performed research; B.K., W.C.L., R.L., – M.D., S.S., M.P., and R.L.-R. analyzed data; and M.D., T.L., R.D., J.D.E., and T.D. wrote formofMPS(MPSI,II,IIIAD, and VII), all associated with the paper. severe neuropathology in affected patients because of massive fl storage of heparan sulfate (and also secondary metabolites) in the The authors declare no con ict of interest. CNS (6). However, the enzymatic removal of two low-abundance This article is a PNAS Direct Submission. sulfate groups, namely 2-O-sulfate from glucuronic acid and 3-O- 1W.C.L., R.L., and M.D. contributed equally to this work. sulfate from glucosamine, have remained enigmatic (7, 8), and 2To whom correspondence should be addressed. E-mail: [email protected]. disorders in which the removal of these sulfate groups is defective This article contains supporting information online at www.pnas.org/lookup/suppl/doi:10. have not been reported. 1073/pnas.1202071109/-/DCSupplemental. 10310–10315 | PNAS | June 26, 2012 | vol. 109 | no. 26 www.pnas.org/cgi/doi/10.1073/pnas.1202071109 Downloaded by guest on September 26, 2021 AB G G‘ toluidine blue 10 µm toluidine blue 10 µm αCalbindin WT Arsg KO CD H H‘ PAS 10 µm TEM 2.5 µm αCD68 WT Arsg KO E E‘ I I‘ autofluorescenceautofluorescence WT 250 µm Arsg KO αCD68 WT 50 µm Arsg KO FF‘ JJ‘ αLamp1 autofluorescence WT 10 µm Arsg KO αGFAP WT 100 µm Arsg KO KLOpen Field Open Field MPassive Avoidance NWater maze probe 350 70 50 16 WT WT WT WT 300 14 Arsg KO 60 Arsg KO Arsg KO Arsg KO 40 250 12 50 * * 10 40 200 30 8 30 150 6 * 20 20 * 100 4 10 Ti me i n Cen ter [s ] 50 2 10 Step through Latency [s] % Path Length in Center 0 0 0 0 3 months 12 months 3 months 12 months Training Test Training Test [s] in Quadrant Time Target 3 months 12 months 3 months 12 months Fig. 1. Neuropathology of Arsg-deficient mice. (A and B) Toluidine blue-stained Epon-embedded sections of the CNS reveal storage vacuoles (arrows) in Purkinje cells (A) and perivascular macrophages (B). (C) Storage vacuoles in both Purkinje cells (long arrow) and macrophages (short arrows) show positive periodic acid–Schiff (PAS) staining. (D) TEM reveals heterogeneous, partially water-soluble, and finely granular to lamellar storage material with variable electron density in Purkinje cells. (E and E′) Fluorescence microscopy of unstained sections shows autofluorescence in the cerebellar cortex of Arsg-deficient mice but not in WT animals. (F and F′) Autofluorescent storage material (red) colocalizes with ring-like structures of the lysosomal membrane protein Lamp1 (green), indicating its lysosomal origin. (G and G′) Considerable loss of Purkinje cells can be observed by 12 mo of age in Arsg-deficient mice as assessed by Calbindin immunohistochemistry. (Inset) Remaining Purkinje cells show swollen dendrites (arrows) and somata (arrowhead). (H–J′) Profound activation of microglia transforming to an amoeboid to phagocytic morphology (H and H′,Iand I′) and hypertrophy of astrocytes (J and J′) occur in Arsg-deficient animals in the cerebellar cortex as assessed with markers for microglia/macrophages (CD68) and astrocytes (GFAP) (age 12 mo). (K and L) Arsg-deficient mice showed a progressive exploratory deficit in the open-field test as indicated by reduced distance traveled (K) and time spent (L) in the center. (M) Lower step-through latencies indicate impaired passive-avoidance learning in Arsg-deficient mice. (N) Water-maze probe trial shows impaired visuo-spatial memory for the platform location in Arsg-deficient mice. For K–N, n = 20, age 12 mo; error bars indicate SEM. fi Behavioral De cits. Behavioral assays revealed that Arsg-knockout (Fig.
Details
-
File Typepdf
-
Upload Time-
-
Content LanguagesEnglish
-
Upload UserAnonymous/Not logged-in
-
File Pages6 Page
-
File Size-